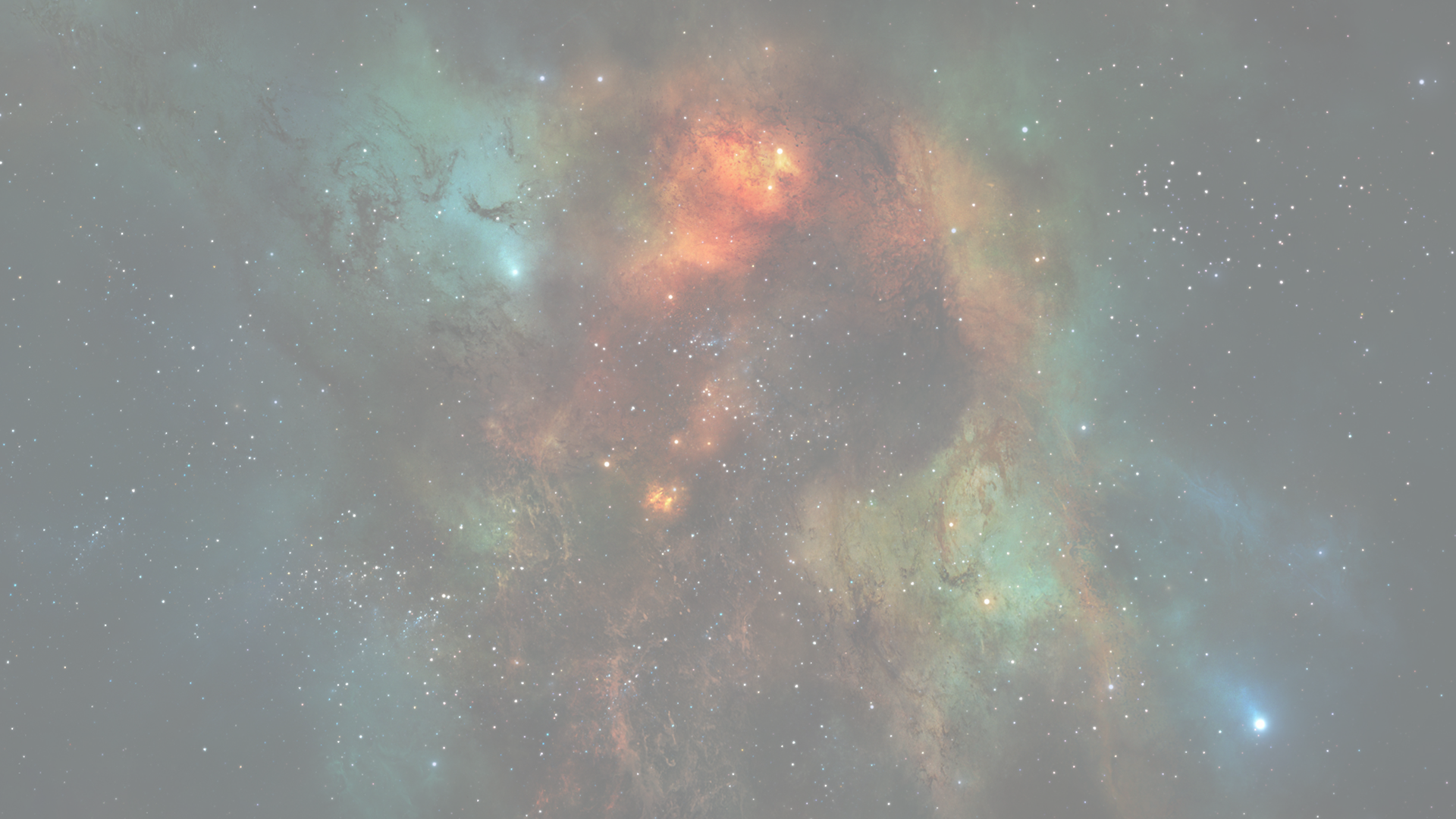
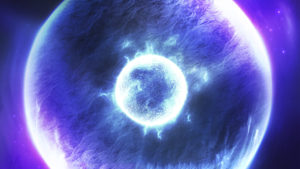
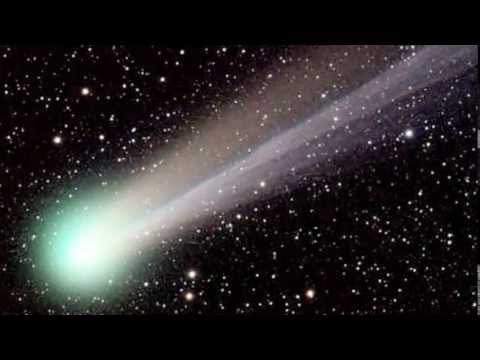
Comets and Coronal Mass Ejections
When a coronal mass ejection greeted Comet NEAT, space scientists called it a spectacular “coincidence.” But in an electric uni- verse such events deserve a second look.
In 2003, as comet NEAT raced through the extended solar atmosphere, a large coronal mass ejection (CME) exploded from the Sun and appeared to strike the comet, causing a ‘kink’ to propagate down the comet’s tail (see lower left). Of course, for solar physicists, the timing of the mass ejection could have no connection to the approach of the comet. However, SOHO has recorded several instances of comets plunging into the solar corona in ‘coincidental’ association with CMEs.
But how would an electric Sun respond to the approach of a relatively small but strongly charged object? In electrical terms, the influence of the comet could be far more significant than its trivial mass in relation to the Sun. Alfvén considered CMEs to be caused by a breakdown or breach of the Sun’s double layer—an event that provokes an explosive exchange between the insulating plasma cell of the Sun and that of the comet. Hence, it not unreasonable at all to ask if a collision of a comet’s sheath with that of the Sun would cause a ‘short-circuit’ that could trigger such an explosion.
When Comets Break Apart
As Comet Linear passed its closest distance to the Sun, it was at its brightest and a prominent dust tail had appeared. Suddenly it fragmented into ‘mini-comets’ (see facing page). Astronomers could find no good reason for its explosive demise. External heat, warming a kilometers-wide chunk of ice, will produce sublimation of the ice but will have virtually no effect a few inches beneath the surface. Many comet watchers began to consider seriously whether comets are actually loosely aggregated collections of ‘mini-comets’ that fly apart when disturbed. But the prior picture of Halley, reinforced by the subsequent close-ups of Borrelly, Wild 2 and Tempel 1, clearly refutes this idea.
At the opposite extreme, Comet West never ap- proached closer than 30 million kilometers to the Sun (half the distance of Mercury). So astronomers were shocked when, in 1976, the comet split into four fragments. Comet Wirtanen fragmented in 1957 a little inside the orbit of Saturn, and something similar occurred to Comet Biela/ Lambert. In fact, eighty percent of comets that split do so when they are far from the Sun, according to Carl Sagan and Anne Druyan in their book Comet.
In a paper published in the 1960s, Dr. Brian G. Marsden, an astronomer at the Smithsonian Astrophysical Observatory in Cambridge, Massachusetts, drew attention to the anomaly of comet fragmentation.110 Discussing the
‘sun-grazing’ comets, he noted that two instances, 1882 II and 1965 VIII, looked as if they had split apart near aphelion (their farthest distance from the Sun), well beyond the orbit of Neptune and far above the ecliptic plane. Moreover, the relative velocity of debris separation was far greater than could be due to solar heating. “One really does require an explanation when the velocity of separation is some 20% of the velocity of the comet itself!” [Emphasis in original paper.]
Such energetic behavior, however, may be expected of an electric comet. Discharges within a comet nucleus are equivalent to the subterranean lightning that causes earthquakes—and just as unpredictable. The resultant ‘comet quake’ has equivalent destructive power and causes the comet to fragment.
According to Sagan and Druyan, “the [splitting] problem is left unsolved.” But they appear to have found a clue without recognizing its significance. “Splitting and jetting may be connected.… At the moment Comet West split, the individual fragments brightened noticeably, and propelled large quantities of dust into space in the first of some dozen bursts.”111 The same could be said for the more recent Comet Linear breakup.
Sudden brightening and explosions of dust are expected to accompany the electrical fragmentation of a comet nucleus as charge is exchanged more furiously with the solar wind over a greater total surface area.
The more sudden the change in a comet’s electrical environment, the more likely that flaring and fragmentation will occur. As we earlier noted, NASA scientists were astonished to observe a remarkable 300,000 km wide flare-up of comet Halley between the orbits of Saturn and Uranus. (Under the assumptions of the ‘snowball’ theory,
Some comments that accompanied this image of the fragments of Comet Linear were, “it was hard to imagine how an object the size of a mountain could totally disintegrate in only two weeks.” And, “The amount of heat available from sunlight just isn't enough to boil away something the size of a mountain in so short a time."
Credit: NASA, H. Weaver (the Johns Hopkins University), and the HST Comet LINEAR Investigation Team See: hubblesite.org/gallery/album/ solar_system_collection/pr2000027b/
110 B. G. Marsden, “The Sungrazing Comet Group,” Astronomical Journal, Vol. 72, p. 1170, 1967.
111 C. Sagan & A. Druyan, COMET, pp. 246-7.
the nucleus should be frozen and inert at that distance.) But the event was no accident. It followed some of the largest solar flares ever recorded.
At the nearest point in its orbit to the Sun, a comet nucleus suffers the maximum electrical stress. This usually results in an increase in brightness of the nucleus due to a larger number of cathode arcs operating simultaneously and more powerfully, explosively removing more solid material into space to form the dust and ion tails. Both of these conditions were noted in the case of Comet Linear, suggesting that the comet was progressing toward an internal discharge.
So it is not surprising to find that fragmentation of comet nuclei is a common occurrence for long-period comets crossing the plane of the ecliptic—where the Sun’s current density is highest in the solar wind. They break up not because they are chunks of ice ‘warming’ in the Sun, and not because they are aggregations of smaller bodies, but because of electrical discharges within the nucleus itself.
This infrared image from NASA's Spitzer Space Telescope shows the broken Comet 73P/Schwassman- Wachmann 3 skimming along a trail of debris left during its multiple trips around the sun. The flame-like objects are the comet's fragments and their tails, while the dusty comet trail is the line bridging the fragments.
Credit: NASA/JPL-Caltech/W. Reach (SSC/Caltech)
Comet Schwassmann-Wachmann 3
Schwassmann-Wachmann 3 provides a case study in electrical fragmentation. The comet was first observed in 1930 and named after its two German discoverers. It completes an orbit every 5.4 years, a path that takes it from just beyond the orbit of Jupiter to inside the orbit of Earth. It does not visit the more remote regions of the solar system where the spectacular ‘Great Comets’ spend long periods adjusting in that more negative environment of the Sun’s domain before racing sunward. What Schwassmann-Wachmann 3 does exhibit, however, is a highly elliptical (elongated) orbit, so in electrical terms that means more rapid transit through the Sun’s electric field and more intense electrical stresses inside the comet nucleus than would
be the case were the comet moving on a less eccentric path.
From its discovery until 1995, it was little more than a footnote in comet science. The first appearance of the comet that year was so bright that astronomers hailed it as a new comet. But as it turned out, the newcomer was Schwassmann-Wachmann 3, presenting itself in more glorious dress than ever before, despite the fact that conditions were not favorable. It was 240 million kilometers away but shining hundreds of times more brightly than expected.
In early 1996, astronomers discovered that the comet had fragmented into at least three pieces, an occurrence clearly linked to the spectacular brightening, though no one could say what caused the event. It also appeared that one or more of the pieces were breaking into secondary fragments.
When the comet returned in 2000, it was again brighter than expected, with indications that the disintegration was continuing—or
even accelerating. Then, with its most recent appearance, the best Hubble images showed dozens of fragments, suggesting the possibility of complete dissolution in a single remaining passage around the Sun.
One astronomer offered this explanation of the comet’s fragmentation: “It’s like pouring hot coffee into a glass that’s been in the fridge. The glass shatters from the shock.”112 But that is not a realistic analogy. The comet is a solid heated from the outside, not a shell heated from the inside. Attributing fragmentation to internal heat stress must explain how heat can be transferred rapidly through hundreds of meters of insulating material, something inconceivable even if you ignore the deep freeze through which the comet is moving, with its sunward face continually changing due to rotation.
In addition to citing possible thermal stresses, the Hubble Space Telescope website offers other possibilities as to why comets might disintegrate so explosively: “They can also fly apart from rapid rotation of the nucleus, or explosively pop apart like corks from champagne bottles due to the outburst of trapped volatile gases.”113 But the centrifugal forces acting on comet nuclei are very small. And to posit heating in the middle of a kilometers-wide dirty ice
cube is, again, scientifically indefensible.
Perhaps, then, Schwassmann-Wachmann 3 “was shattered by a hit from a small interplanetary boulder,”114 offered one of the astronomers quoted above. “Well, make that a series of one-in-a-trillion hits,” mused a critic of today’s comet science. “That way we can explain the continuing fragmentation over years.”
When Asteroids Become Comets
According to recent scientific reports, astronomers are “rethinking long-held beliefs about the distant domains of comets and asteroids, abodes they’ve always considered light- years apart.” The discovery of asteroids sporting comas has forced astronomers to speculate that some asteroids are actually “dirty snowballs in disguise.”
For many years the standard view of asteroids asserted that they are composed of dust, rock, and metal and that most occupy a belt between Mars and Jupiter. In contrast, comets were claimed to arrive from a home in deep space, most coming from the imagined ‘Oort Cloud’ at the outermost reaches of the solar system.
But now, “the locales of comets and asteroids may not be such a key distinction,” states Dan Vergano, reporting on the work of two
112www.smh.com.au/news/world/comets-breakup-has-scientists-ringside-for-show-of-
a-lifetime/2006/04/30/1146335611925.html
113 hubblesite.org/newscenter/archive/releases/2006/18/image/a
114 science.nasa.gov/headlines/y2006/24mar_73p.htm
Orbits of the three known main-belt comets (red lines), the five innermost planets (black lines: from the center outward; Mercury, Venus, Earth, Mars, and Jupiter), a sample of 100 main-belt asteroids (orange lines), and two ‘typical’ comets (Halley’s Comet, and Tempel 1, target of the recent Deep Impact mission) as blue lines. Positions of the main-belt comets and planets on March 1, 2006, are plotted with black dots.
Image credit: Pedro Lacerda (Univ. Hawaii; Univ. Coimbra, Portugal)
University of Hawaii astronomers, Henry Hsieh and David Jewitt.115 In a survey of 300 asteroids lurking in the asteroid belt, the astronomers detected three objects that “look a lot like comets …
ejecting little comet tails at times from their surfaces.”116 The three red circles in the illustration on the previous page describe the orbits of these ‘comet-like’ asteroids. One large (140 km) object, Chiron, mentioned at the beginning of this chapter, is classified as both an asteroid and a comet. Chiron’s orbit is highly eccentric, with perihelion just inside the orbit of Saturn and aphelion just inside the orbit of Uranus.
In the electric view, there is no real distinction between a comet and an asteroid, apart from their orbits. Thus, both Chiron and the illustration make the point for us: the red circles show greater variations in orbital distances from the Sun.
Comet Shoemaker-Levy 9
The famous collisions of fragments of Comet Shoemaker-Levy 9 (SL-9) with Jupiter provide a spec-
tacular confirmation that comets have a store of energy in addition to their kinetic energy. On 7 July 1992, SL-9 grazed past the giant planet Jupiter a mere 20,000 km above the cloud tops. It had penetrated deep into Jupiter’s huge plasma sheath. As it switched suddenly from the Sun’s electrical environment to that of Jupiter, it would have experienced extraordinary internal electrical stress. Unsurprisingly, it broke up. In fact, after the main disrup- tion event some of the fragments split further in the rap- idly changing electrical environment. It is this tendency to fragment, when gravitational and rotational forces are far too weak to explain it, that gives rise to the idea ex- pressed by some astronomers that comets are a pile of fragments.
The fragments of SL-9 returned to collide with Jupiter during the week of 16–22 July 1994. Some astronomers predicted that the fragments were too small to have much
effect. “There’s a chance we will see very little,” hedged Eugene Shoemaker, late of the Lowell Observatory in Flagstaff, Arizona, and co-discoverer of the comet, shortly before the event.
Since SL-9 had done nothing to distinguish itself before it broke up— it couldn’t be found in images taken before the break-up—astronomer Brian Marsden surmised that it was 1 to 2 kilometers in diameter. “It’s
115 H. Hsieh & D. Jewitt, “A Population of Comets in the Main Asteroid Belt,” Science, Vol 312, 28 April 2006, pp. 561-3.
116www.usatoday.com/tech/science/columnist/vergano/2006-03-26-comet-
going to be tough to see much,” he concluded. “I don’t think there’s going to be a very large explosion.” But planetary physicist Jay Melosh summed up the uncertainty, “Theoreticians are often wrong, especially in predicting things.”117 As we know, the spectacle exceeded all expectations. But were the collisions simply impacts in a purely mechanical sense—or did the electric charge of the comet fragments contribute significantly to the event?
Initially, the dazzling display baffled astronomers because there were remarkable electrical phenomena. Renée Prange of the French Institute Astrophysique Spatiale, a member of the Hubble upper atmosphere imaging team, saw ‘northern lights.’ Ultraviolet images showed glowing streaks in Jupiter’s northern hemisphere which appeared as almost mirror images of glows from the impact site of fragment ‘G’ in the southern hemisphere. According to Dr. Prange, the northern glows appeared farther south than ever before: “I think it’s a major discovery.” The Jovian auroral displays appeared to be triggered by electrically charged particles released during the impacts in the south following a looping arc northward along the planet’s magnetic field until they fell back into the planet’s atmosphere, creating a glow in the north. According to Dr. Prange, it is still unclear whether the particles were comet dust that became electrically charged as it fell through the planet’s magnetic field or gas molecules from the planet that became charged in the heat of the fragment’s impact and explosion.118
Then, after a year’s analysis “by hundreds of talented scientists,” Nature119 issued a consensus report: “First seen was a faint glow that slowly increased in brightness on a time-scale of tens of seconds, be- lieved to be due to a large number of small meteors in the coma sur- rounding each SL-9 fragment. The meteor shower was followed by a sharp increase in brightness as the main part of the fragment entered the Jovian atmosphere…. A few tens of seconds later, a fireball ex- ploded up the ‘chimney’ created in the atmosphere by the bolide.”
The explanation for the initial glow, coming after the fact, only served to confirm the power of ideology in comet science. In electrical terms, the charged comet fragments would be expected to exhibit a glowing coma as they approached Jupiter. The glow would increase until a sudden arc discharge would occur between Jupiter and the comet fragment. The steep increase in brightness that astronomers observed has more in common with a lightning flash than the light- curve of a bright bolide’s entry into the Earth’s atmosphere. In fact, it
117 R. A. Kerr, Science, Vol. 265, 1 July 1994, pp. 31-2.
118 The Baltimore Sun, 21 July 1994, p. 12A.
119 P. J. T. Leonard, “Impact consensus emerges,” Nature, Vol. 375, 1 June 1995, p. 358.
was expected that the fragments would flare several times like fireballs that enter the Earth’s atmosphere.120 That didn’t happen.
As we shall see, there is no evidence that the fragments entered the atmosphere. That and the fireball exploding up a ‘chimney’ are simply presuppositions of the impact model. And there were many other anomalies for the impact model.
In Jupiter’s plasma environment, we can expect electrical forces to affect the trajectories of the charged comet fragments. Such forces would cause deviations from expected impact times. And in fact impact times were on average 8 minutes later than expected.121
One of the largest pieces, fragment G, showed spectral evidence of magnesium when it was just 10 hours from impact. Such metals only show up when comets graze around the Sun. But whatever was tearing magnesium from the fragment as it sped in through Jupiter’s magnetosphere couldn’t drive off enough water to be detectable. That failure to observe water gave rise to questioning whether the comet might be an asteroid. After all, only the fuzziness of SL-9 identified it as a comet, and some asteroids have been observed occasionally to show fuzziness.122
The electrical comet model explains each and every mystery of SL-9. An electric comet is as dry as an asteroid. The ‘fuzziness’ of a comet is due to electric currents flowing in its plasma sheath, causing the sheath to glow. When the electrical stress increases beyond a threshold, the plasma in the sheath will establish arcs at the surface, which will machine dust and atoms, such as magnesium, from the minerals there. That this should occur shortly before the collision is not surprising: Jupiter’s magnetosphere is the most active electrical environment for a comet outside a close encounter with the Sun.
Dr. Earl Milton, before the above paper was published, wrote, “When comet Shoemaker-Levy 9 meets Jupiter, spectral changes in the comet’s tails might become conspicuous once the comet leaves the solar wind and enters Jupiter’s electrosphere [magnetosphere or plasma sheath]. This part of the encounter precedes by hours the meeting of the nuclei with Jupiter’s atmosphere.”123 Here we see the contrast between an old hypothesis that should be discarded and a better one. The electric comet hypothesis has explanatory and predictive power.
The Galileo spacecraft on its way to Jupiter, the Hubble Space Telescope, and many terrestrial observatories tracked the fragments as
120 Z. Sekanina, “Disintegration Phenomena Expected During Collision of Comet Shoemaker-Levy 9 with Jupiter,” Science Vol. 262, 15 October 1993, pp. 382-3.
121 H. B. Hammel et al, “HST Imaging of Atmospheric Phenomena Created by the Impact of Comet Shoemaker-Levy 9,” Science, Vol. 267, 3 March 1995, p. 1288.
122 Science, Vol. 265, 19 August 1994, p. 1030.
123 E. R. Milton, private correspondence, 24 July 1994.
they approached Jupiter. Their data highlighted another mystery. Some collisions that were calculated to occur just beyond Jupiter’s limb and that should have been invisible to all but the Galileo spacecraft were seen from the Earth. In a NASA news report,124 Dr. Andrew Ingersoll said, “In effect we are apparently seeing something we didn’t think we had any right to see.” “It seems clear that something was happening high enough to be seen beyond the curve of the planet,” said Dr.
Torrence V. Johnson of JPL. The predictable electrical event prior to the fragment striking Jupiter’s upper atmosphere, did indeed occur.
Chemical analyses threw up more mysteries. Sulphur, ammonia, carbon disulphide and glowing acetylene and methane heated by the collisions were found—but no water or even an oxygen-bearing molecule. This is a problem because the current theory of Jupiter’s structure requires a layer of water clouds below the top clouds of ammonia. Present theories of the formation of the Solar System require that both Jupiter and comets have water—yet no one found signs of any water at all.
According to Dr. Lucy McFadden of the University of Maryland, “It is disturbing. This means either that our modeling is not correct, or the comet exploded before it reached Jupiter’s water layers.” But SL-9 was originally classified as a comet because its
fragments each appeared to have a ‘coma,’ assumed to be a halo of water vapor, dust and gas.125 An explosion above the hypothetical water layers of Jupiter would still not explain why none of the comet’s own water turned up. That leaves only the first half of McFadden’s either-or: “our modeling is not correct.”
What, then, were the vertical jets seen rising three thousand kilometers above Jupi- ter’s atmosphere and known as ‘plumes?’ And
what were the crescent-shaped dark features that resulted from the fallback of the plume onto the atmosphere? The dark material came to be known technically as the ‘brown stuff,’ its nature unknown (right).
Melosh suggested that the comet fragments would penetrate Jupiter’s atmosphere so deeply before exploding that they would be swallowed up and we would see very little. Others proposed that each fragment would dig a ‘tunnel of fire’ in Jupiter’s atmosphere before exploding and sending a plume of hot atmospheric and cometary material from the top of the tunnel into space. This was the ‘plume’ model that was explored to try to explain the strange dark fallout pattern.
In this time-sequence image of SL-9 fragment G’s collision with Jupiter. The number is the time from impact in hours. Note the strange ‘rays.’ The wavelengths recorded from left to right are 889 nm [infrared], 555 nm [visible], and 336 nm [ultraviolet]. The infrared image (LEFT) shows the dark material to be warm (bright). North is up; Jovian west longitude increases to the left.
Image Credit: NASA-ESA Hubble Space Telescope, STScI.
Credit: H. B. Hammel et al., HST Imaging of Atmospheric Phenomena Created by the Impact of Comet Shoemaker-Levy 9, Science, Vol. 267,
3 March 1995, p. 1289.
124 www.jpl.nasa.gov/releases/94/release_1994_9465.html
125 Baltimore Evening Sun, 20 July 1994, p. 9A.
A so-called ‘volcano’ on Io shows the typical penumbral fallout ring of a plasma gun. It is a precise analog of the fallout rings on Jupiter generated by plasma arcs between Jupiter’s iono- sphere and the comet fragments.
Image credit: JPL & NASA
However, the plume model could not explain the clear zone be- tween the dark core and the crescent. Nor could it explain the radial lines dissecting the crescent.
Ironically, the answers to the puzzles come from Jupiter’s closest moon, Io. In November 1979, the noted astrophysicist Thomas Gold proposed that the gigantic plumes on Io are not volcanic but evidence of electrical discharging.126 Years later, a paper by Peratt and Alex Dessler followed up Gold’s suggestion, showing that the discharges took the form of a ‘plasma gun effect,’ which produces a parabolic plume profile, filamentation of the matter within the plume, and the termination of the plume onto a thin annular ring.127 These are precisely the effects seen in the encounter of SL-9 with Jupiter.
All of the unexplained oddities begin to make sense, if a plasma discharge occurred between the highly charged comet fragments and Jupiter’s ionosphere. The electromagnetic pinch of the plasma gun effect preferentially heats ions to temperatures far hotter than the Sun and produces the bright, lightning-like flash and subsequent glow. That is why the light from fragments that were expected to impact beyond the limb was unexpectedly visible. The light from a discharge at 3,000 km above Jupiter’s cloud tops would have been visible from Earth.
The electrical nature of the event will also explain why comet fragments of different sizes created fireballs of the same height.128 The discharges occurred as different fragments penetrated the same ‘double layer’ of Jupiter’s plasma sheath.
A plasma discharge would also explain why the expected com- pounds, such as water, from deeper cloud layers were not seen in the plumes. The comet fragment is vaporized and ionized by the energy of the discharge. Constrained by powerful electromagnetic forces, the silicate particles and other ionized compounds from the rocky comet form the warm plume and crescent-shaped fallout pattern of ‘brown stuff.’
What was the ‘brown stuff?’ The Hubble Space Telescope found “most surprising were the strong signatures from sulfur-bearing compounds like diatomic sulfur (S2)...”129 However, S2 is a molecule with a very short lifetime and its origin is unknown. “The origin of sulfur in ..comets remains enigmatic.”130 If we return to Io, we find that the ‘plasma gun’ effect there has covered the moon with colorful
126 T. Gold, “Electrical Origin of the Outbursts on Io,” Science, Vol. 206, 30 November 1979, pp. 1071-3.
127 A. L. Peratt, A. J. Dessler, “Filamentation of Volcanic Plumes on the Jovian Satel- lite Io,” Astrophysics and Space Science 144 (1988) pp. 451-61.
128 Sky & Telescope News, “Astronomers discuss Comet Crash,” November 4, 1994.
129 NASA News Release 94-161, “Hubble Observations Shed New Light on Jupiter Collision,” p. 3.2.
130 J. Crovisier & T. Encrenaz, Comet Science, p. 49.
sulfur molecules, including red and brown variants. A large quantity of oxygen was observed in the SL-9 events at Jupiter. So it seems likely that the sulfur was formed by fusing two oxygen atoms together in the powerful plasma discharges to form one sulfur atom. Originally, Io was probably an icy moon like its Galilean siblings.
There was no water from the comet, and Jupiter’s deeper cloud layers, if they did contain water, were not pulled into the plume.
Jupiter’s magnetic field is probably responsible for most of the rotation, asymmetry, and offset of the plasma gun discharge pattern.
It may be that children listening on their school’s radio telescope provided the most significant observation of all: they said that the SL-9 collisions were accompanied by bursts of radio emissions “just like those from sunspot activity on the sun.”131
The New Scientist called this “Jupiter’s surprise radio broadcast.”132 Astronomers had expected radio emissions at high frequencies to diminish and to hear the comet crash clearly at low frequencies. Instead, nothing happened at low frequencies while emissions around 2–3 gigahertz rose by 20 to 30%. “Never in 23 years of Jupiter observations have we seen such a rapid and intense increase in radio emission,” said Michael Klein of JPL. The radio emission peaked on 23 July, just after the last comet fragment hit, and it declined thereafter. Klein had expected dust from the comet to absorb electrons, which otherwise might contribute to radio emissions. “Instead, extra electrons were supplied by a source which, as yet, is a mystery.” But it is no mystery. Like all comets, SL-9 was negatively charged, its fragments supplying copious electrons for the radio emissions.
The fate of SL-9 thus provides a consistent picture of the electric comet. The picture includes the original break-up and and subsequent further fragmentation of the comet, plus the surprising events that occurred in the 1994 impact with Jupiter: the bright plumes above the Jovian atmosphere; the sightings of ‘impossibly’ energetic events from Earth; the associated Jovian auroral displays; the absence of water in the vaporized debris; and the lack of the expected constituents from Jupiter’s atmosphere, all pointing to the termination of each fragment’s flight in spectacular flashes before it entered Jupiter’s atmosphere.
The famous ‘string of pearls’ of comet Shoemaker-Levy 9 frag- ments before impact.
Credit: NASA, Hubble Space Telescope (courtesy of H. Weaver)
131 BBC Radio 4 Science Now, 19 July 1994.
132 New Scientist, 20th August 1994, p. 17.
Comet Tempel 1 67 seconds after it obliterated Deep Impact’s projectile. Light from the collision saturated the camera’s detector. Scalloped craters can be seen.
Image Credit: NASA/JPL-Caltech/UMD
The white areas on comet Tempel 1 are the cometary equivalent to “St. Elmo’s fire” – coronal glow discharges some- times observed dancing on high points in lightning storms on Earth. Similar, but more powerful arcs on Jupiter’s moon Io produced ‘whiteouts’ that overloaded the Galileo probe camera and surprised the investigators.
Image Credit: NASA/JPL--Caltech/UMD
Deep Impact: The Smoking Gun
On July 4, 2005, one of the most watched events of the space age occurred, when an 820 pound copper projectile, fired from the Deep Impact spacecraft, struck the nucleus of Comet Tempel 1. The following is a partial summary of correct predictions for Deep Impact based on the electric comet model. Many of these predictions were made as early as October, 2001, and some on the eve of the event.133
- Energy of Explosion. “More energy will be released than expected because of the electrical contributions of the comet.” It is now well documented that the scale of the energetic outburst stunned every scientist associated with the project. These scientists knew the kinetics of impact, and they all agreed that the explosion would be equivalent
to 4.8 tons of TNT. That’s a good-sized bomb, but not even close to what occurred.
- Advance One of the authors, Wallace Thornhill, predicted at least one flash from electric discharge prior to impact. From the standard viewpoint, that is an absurd prediction when considering an impactor hitting a body at 37,000 km per hour in ‘empty’ space. But here is NASA investigator Peter Schultz’s description of the event: “What you see is something really surprising. First, there is a small flash, then there’s a delay, then there’s a big flash and the whole thing breaks loose.”
- Missing “An abundance of water on or below the surface of the nucleus (the underlying assumption of the ‘dirty snowball’ hypothesis) is unlikely.” “It’s pretty clear that this event did not produce a gusher,” said SWAS principal investigator Gary Melnick of the Harvard-Smithsonian Center for Astrophysics. “The more optimistic predictions for water output from the impact haven’t materialized….” (See information panel p. 94.)
- Sharp Surface “The model predicts a sculpted surface, distinguished by sharply defined craters, valleys, mesas, and ridges.” All of the expected features are present, and astronomers cannot agree on the cause, though all agree that Tempel 1 does not look like a melting snowball.
- Surface Arcing. This prediction is, of course, inherent in the electric model. The highest resolution photographs of Tempel 1, taken by the impactor, show numerous unresolved and unexplained white spots (left), most located where the electrical hypothesis would put them—on the rims of craters and on the wall of cliffs rising above flat valley Electrical etching continually expands valley floors by eating away at the sharp edges of surrounding cliffs.
133 See: www.thunderbolts.info/tpod/2005/arch05/050704predictions.htm
- New Jets. Thornhill was the only one to have anticipated a shift in the arrangement, number, and the intensities of the jets away from the impact site. The 2.5-meter NOT telescope of the El Roque de los Muchachos observatory at La Palma, Spain released images just before impact and 15 hours after impact. The observatory report states, “New jets appeared after the ” No explanation has been given.
- Electrostatic Prediction: “electrical arcing and ‘electrostatic cleaning’ will clean the nucleus’ surface, leaving little or no dust or debris on it.” The surface of Tempel 1 contrasts with the
surface of the asteroid Itokawa (see following page). The asteroid appears to have attracted considerable surface debris electrostatically. We suggested an active comet would do the reverse. Removal of surface debris would then be analogous to the ‘miraculous’ cleaning of the solar panels of the Mars rover Opportunity.134
Deep Impact and Missing Water
Through much of the space age, comet investigators have been hoping to confirm the presence of water on comet nuclei. But it seems that comets have been unwilling to cooperate.
By the time of Deep Impact comet theory had fragmented into contradictory hypotheses, due in part to the lack of detectable water on cometary surfaces—a prerequisite of comet theory.
In 1986, visits to comet Halley by the European Giotto and Rus- sian Vega probes failed to locate surface water and raised the distinct possibility that the nucleus might not be ejecting water into space.
In January 2004, the Stardust spacecraft passed by Comet Wild 2, identifying a dozen jets of material exploding from the nucleus. The craft plowed through surprisingly dense pockets of dust swirling around the comet, but investigators were astonished that they could not find even a trace of water on the surface, despite the energetic activity.
According to a NASA report, the flyby of Comet Borrelly by the Deep Space 1 craft in 2001 “detected no frozen water on its surface.”
When comet Shoemaker-Levy 9 broke apart, astronomers reasoned that the fractured nucleus would expose fresh ices that would sublimate furiously. So several ground-based telescopes and the Hubble Space Telescope trained their spectroscopes on the tails of the fragments of SL-9, looking for traces of volatile gases. None of the gases was found.
134 www.thunderbolts.info/tpod/2005/arch05/050531roverclean.htm
When Comet Linear disintegrated in front of their eyes, astronomers were not just shocked by the event (a comet exploding many millions of kilometers from the Sun), they were astonished to find virtually no water in the immediate debris.
The absence of detectable water on comet nuclei had produced a crisis in comet theory well before Deep Impact. And that mission did nothing to rescue the theory. The Harvard-Smithsonian Center for Astrophysics summarized the early findings with the headline, “Deep Impact Was a Dust-up, Not a Gusher.” Smithsonian astronomers reported “only weak emission from water vapor and a host of other gases that were expected to erupt from the impact site. The most conspicuous feature of the blast was brightening due to sunlight scattered by the ejected dust.”
The results of the Deep Impact mission were published in the journal Science. Team members reported that they found only a smattering of water ice on the surface of Tempel 1. To account for the water supposedly emitted into the coma of Tempel 1, the investigators needed 200 times more exposed water ice than they could find. The electrical alternative faces no such dilemma (see information panel p. 94).
Image of the asteroid Itokawa (25143), taken by Japan’s probe Hayabusa. Asteroid Itokawa demonstrates the difference between the surface of a rocky body that has not suffered electric arc machining or ‘electrostatic cleaning’ like comet Tempel1.
Credit: JAXA
Comet Origins
Plasma discharge phenomena are scalable over more than 14 orders of magnitude.135 This means that phenomena seen in the laboratory and recorded in billionths of a second may span light-years in deep space and could last for centuries or more. This scalability of plasma phenomena enables us to compare the detailed structures of high-energy laboratory discharges with galaxy-sized events in space.
In the Electric Universe model, the solar system has a dynamic history, unimagined in any prior cosmogony. In our first volume, Thunderbolts of the Gods, we provide abundant forensic evidence that the present order in the solar system
was established shortly before the rise of civilization. We observed that archaic references to comets describe them as being born in the close approach of planets when “the space between the two planets lights up and is set aflame by both planets and produces a train of fire.”136 The description makes clear that this was no modern ‘conjunction’ of remote planets where their bright points of light merely appear close together in the sky.
Instead of conjecture, we have compelling forensic evidence of the origin of the comets, asteroids and meteoric rocks. They were all
135 A. L., Peratt, Physics of the Plasma Universe, (Springer-Verlag) 1992, p. 48.
136 Seneca, Naturales Quaestiones, 7. 12. 1, transl. Corcoran II 1972: 250-51.
born in the violence of planetary electrical encounters. From this conclusion, we begin to understand why Mars, the god of war, is the source of meteorites that still arrive on Earth. Astronomer Tom Van Flandern has advanced cogent arguments “that comets originated in the energetic breakup of a body orbiting the Sun in or near the present location of the asteroid belt in the relatively recent past.”137 However his view that a ‘nova’ type explosion of a former planet was responsible begs the question of what would cause such an outburst. In the Electric Universe, a nova is a stellar manifestation of electric discharge. Consequently, the orbits of comets favor an origin in an electrical ‘planetary nova’ event.
A New Perspective
“There is no such thing as consensus science. If it's consensus, it isn't science. If it's science, it isn't consensus. Period.”138
From the evidence briefly noted here, we conclude that the consensus about comets can no longer be maintained. Though the story has been presented as fact for several decades, a new perspective is emerging. A comet is an electrical phenomenon, as intuited by 19th century scientists. The immense envelope of the coma, perhaps several million kilometers across and bigger than the Sun, is a plasma sheath enclosing an electrified body. The filamentary comet ion tails, stretching across the sky, are part of the circuit carrying current from the comet. Given the fundamental electrical nature of the Sun and interplanetary space, a comet is subject to electrical transactions far more energetic and complex than expected.
To state the point bluntly, the underpinnings of modern comet theory have collapsed, though no official announcement of this fact has been forthcoming. Nor has official science yet faced the implications for planetary history. If cometary displays are electrical discharges, then a planet on an elliptical orbit will suffer proportionate electrical stress. It will become a comet and suffer surface etching. Certain objects seen in deep space take on new interest as well, because it is possible to see them as scaled up analogues of a comet.
Indeed, it appears that the Gemini Observatory
using adaptive optics has seen stellar scale ‘comets’ far from our solar system in the Orion nebula (right). And the comet-like formations seen
137 T. Van Flandern, Dark Matter, Missing Planets & New Comets, pp. 185-6. “[Asteroid] orbits exhibit ‘explosion signatures,’ which are a set of characteristics in the distribution of orbits that imply origin in an explosion.”
138 M. Crichton, "Aliens Cause Global Warming," a lecture at CalTech, Pasadena, CA, January 17, 2003.
The image shows the Orion "bullets" as blue features and represents the light emitted by hot iron gas. The light from the wakes, shown in orange, is from excited hydrogen gas. The typical size of one of the bullet tips is about 10 times the size of Pluto's orbit around the sun. The wakes shown in the image are about a fifth of a light-year long.
Credit: Gemini Observatory
in the famous Cartwheel galaxy (left), which may be cometary electrical activity on a galactic scale.
Illustration of comet-like objects racing at more than 1 million km/hr through the Cartwheel Galaxy's center. The ‘heads’ are a few hundred light-years across; the tails are several thousand light-years long. The string of bright lights in the background is the galaxy's outer ring of young stars. The galaxy is in the constellation Sculptor.
Credit: J. Gitlin (Space Telescope Science Institute), and NASA
Looking Past the Familiar Boundaries
If there is a single message in the evidence we have summarized here, it is that specialists must pause to hear witnesses from outside their own disciplines. It is not customary for the experts on comets to consider the physics of the Sun. Nor do planetary geologists normally show interest in the study of lightning. Cosmologists give little attention to the behavior of auroras.
As theoretical challenges arise, the tendency is to look for solu- tions within one’s accustomed frame of reference. But will this habit really serve our interests when the problem lies with our fundamental assumptions? In the nineteenth and early twentieth centuries, certain notions came to be codified within the theoretical sciences, as if the principles were self-evident and could not be questioned—the rule of gravity, the stable and predictable solar system, and the electrically neutral cosmos. Yet many of the challenges to these suppositions come from outside any particular specialty. If a foundational assumption is challenged by unexpected patterns of discovery, a predicament will arise. Within the different fields, the specialists will not realize that the most important clues could lie outside their familiar subject.
If lightning and auroras involve electric interactions of the Earth and the Sun, then space plasma is not electrically inert and cosmologists’ faith in gravitational models is misplaced. If the Io plumes are electrical discharges, then planetary geology must be rewritten. If a comet is discharging electrically as it approaches the Sun, then the Sun is the center of a radial electric field, though solar physicists have never entertained the possibility.
Similarly, if distinctive surface features of solid planets, moons, and lesser rocks in the solar system were created electrically, then long-standing assumptions about planetary stability are mistaken, and the implications will reach far beyond planetary geology. All inquiry into the Earth’s history will be affected.
Abell 754 |
23-4 |
|
æther |
22 |
|
accretion disk |
25, 92 |
|
Adams, D. |
2 |
|
Alfvén, H. |
3, 6, 9-12, 23-7, 33-5, 46-7, 59, 63-4, 66, 68, 85, 90, 94, 102, 104 |
|
Ampère, A. M. |
7 |
|
Arp, H. C. |
13-20, 23 |
|
aurora |
5, 7-10, 12, 29, 32-3, 39, 42, 46-8, 51, 53, 85, 96, 109, 113, 118 |
|
Bering, E. |
48-9 |
|
Betelgeuse |
80-1 |
|
Big Bang |
1, 2, 11-6, 18-21, 23-4, 27, 34 |
|
Birkeland, K. R. |
7-10, 12, 33, 35, 43, 46-7, 53, 56, 61, 72, 85 |
|
birkeland current |
7-8, 12, 26-7, 34-6, 38-40, 55, 61, 69, 72, 83, 93 |
|
black hole |
2-3, 20, 22, 25, 26, 28, 34 |
|
Bostik, W. H. |
28 |
|
bow shock |
47, 52, 68, 102 |
|
brown dwarf |
84 |
|
Burbidge, G. |
14-5, 18, 24 |
|
capacitor |
6, 49, 51, 95, 97 |
|
Chandrasekhar, S. |
25 |
|
Chapman, S. |
1, 33, 56 |
|
chromosphere |
45, 62-3, 65-7 |
|
CMBR (Cosmic Microwave Background Radiation) |
14-5 |
|
Cobine, J. D. |
57 |
|
comet |
29, 35, 85-95, 97-118 |
|
Biela/Lambert |
105 |
|
Borrelly |
29, 88, 97, 99, 115 |
|
coma 29, 43, 86, 88, 90, 92-5, 101-3, 107, 109, 111, 116-7
Halley 88, 90, 95, 101, 103-5, 107, 115
Hyakutake 103
Linear 97, 103-6, 116
Shoemaker -Levy 9 108-13, 115-6
Tempel 1 88, 90-1, 94, 97, 104, 107, 114-6
West 93, 104-5
Wirtanen 104
corona 44-5, 53, 60, 62, 65-7, 78, 104
Crab nebula 24
Crookes, W. 41
Cygnus Loop 39
dark energy 24
dark matter 2-3, 19, 22, 24, 26, 36, 89, 117
Deep impact 90-1, 94, 99, 107, 114-6
Dicke, R. 14
double layer 9-10, 40, 46, 59, 64, 73, 80, 104, 112
Earth 4-5, 7-13, 16, 18-9, 21-2, 30-3, 35, 39-49, 51, 55-6, 58, 64
66-8, 70, 73, 76, 82-4, 88-9, 95, 97-9, 105-7, 109, 111-3, 117-8
Einstein, A. 2, 3, 21-3, 27, 35
electret 95
electric discharge machining (EDM) 88, 93, 99-100
Feynman, R. 31
Finlay-Freundlich, E. 14
fractal universe 27
Franklin, B. 5-7
fusion 12, 53-4, 60, 62-3, 70, 73, 76
Galaxy 0313-192 25
Galileo spacecraft 42, 88, 110
Gamow, G. 14-5
Geissler tube 59
glow discharge 34, 41, 47, 49-50, 56-60, 67-8, 80, 89, 93, 96
Gold, T. 45, 112
gravity 1-3, 5, 8, 13, 21-6, 28, 30-2, 36-7, 46, 53, 70, 77, 86, 89, 95, 97, 99, 118
GRB (Gamma Ray Burst) 16-17
Guillaume, C. 14-15
Guth, A. 2
heliosphere 44-5, 57, 67-8
Herbig Haro object (HH-34) |
27 |
Herbig Haro (HH-111) |
36 |
Hertzsprung-Russell (H-R) diagram |
73-4 |
Hooke, R. |
4 |
Hoyle, F. |
2, 14-5, 25-6, 70 |
Io |
52, 88, 112-3 |
jet 17, 24-5, 27-8, 35-6, 40, 48, 52, 54, 86, 88, 92-4, 97, 100-2, 104, 111, 115
Juergens, R. 33, 56-9, 63, 65, 67, 78, 100
Helix nebula 38
Hubble, E. 13
Huchra, J. 14
Jammer, M. 21
Jansky, K. 39
Jupiter 23, 33, 41-2, 47, 50, 52, 84, 88, 92, 106-13
Klein, M. 113
Langmuir, I. 8-9, 12, 41, 44, 49, 89
sheath 9, 41, 43-7, 50, 97
probe 12, 41
Lightning 1, 5-6, 27, 30, 32, 42, 46-51, 55-6, 66, 70, 72-3, 86, 96, 105, 109, 112, 118
McKellar, A. 14
Magnetohydrodynamics 9, 12, 27, 35, 52, 90
Magnetosphere 8-9, 12, 30, 33, 38, 41, 43, 45, 47-8, 58, 64, 82, 110
Mars 42, 100, 107, 115, 117
Mather, J. 14
Menzel , D. 6
Milky Way 1, 15, 23, 27, 68, 72, 82, 84
Millikan, R. 31
Milton, E. 100, 110
Moon 5, 40-1, 94, 100
M51 36; M81 16; M87 24-5, 28
Neutron star 34, 82
Newton, I. 2, 3-5, 21-3, 35-6, 38
NGC 1313 19; NGC 309 16; NGC 4458 34; NGC 6543 Cover;
NGC 6826 40; NGC 7319 17, 20 |
|
Peratt, A. L. |
9, 23, 26, 30, 82, 112, 116 |
photosphere |
45, 53-6, 58-9, 60-3, 65-7, 70, 71, 75, 78 |
Pioneer spacecraft |
36, 41-2, 57 |
planet, planetary |
1, 3-5, 7, 9, 11-2, 22, 30, 35-41, 43-9, 52-4, 56-8, 67, 68 |
70, 72, 84-6, 88-9, 91-2, 98-9, 101, 107-9, 111, 116-8 |
|
plasma focus |
37 |
cosmology |
11-2, 17-8, 34-5, 61, 68 |
pulsar |
82 |
quasar |
13-4,17-20 |
radio |
1, 7, 9, 15, 17, 24-5, 27, 34, 39, 84, 113 |
red dwarf |
75 |
red giant |
74, 76-8, 80-2 |
redshift |
13, 16-20, 24 |
Regener, E. |
14 |
relativity |
3, 22 |
Sagan, C. |
20, 105 |
Saturn |
5, 88, 105, 108 |
Scott, D. E. |
i, 72, 74-5 |
Shmaonov, T. |
14 |
solar system |
3-4, 8, 10, 15, 23, 33, 36, 44, 51, 56-7, 61, 65 |
68, 72, 84-6, 88-94, 98-9, 106-7, 111, 116-8 |
|
Space Shuttle Columbia |
42 |
sprites |
48-51 |
Stardust mission |
85, 98-101, 115 |
Stephan’s Quintet |
17, 20 |
string theory |
2, 21 |
sun 3, 5-8, 12, 22-3, 30, 33, 36, 38, 41, 43-9, 53-7981, 84-9, 91-5, 97-100, 103-6
108-10, 112-3, 116-8
sunspot 7, 53-5, 58, 61, 63, 65, 70-,3, 75, 79, 85, 113
supergiant 80-2
Terrella 7-8, 10, 12, 33, 43, 46-7, 61, 72, 85
Tethered Satellite System (TSS) 42
tornado 55, 66-7, 72, 84
Turner, M. 14
Thornhill, W. 4, 14, 30, 50-1, 65, 68, 84, 100, 114-5
universe
Uranus 88, 105, 108
ULIRG (Ultra Luminous Infra Red Galaxy) 16
ULX (Ultra Luminous X-ray source) 17-9
Van Allen, J. 8, 43, 50
Van Flandern, T. 20, 89, 117
Venus 41, 50, 102, 107
Verschuur, G. 15
Virgo cluster 18-9
water 11-2, 17, 50, 52, 71, 84, 86, 91, 93-5, 97-8, 101-2, 110-6
white dwarf 74, 76-80
WMAP (Wilkinson Microwave Anisotropy Probe) 2, 15 Z-pinch 32, 35, 50, 69, 82-3, 92