“[Those] who have an excessive faith in their theories or in their ideas are not only poorly disposed to make discoveries, but they also make very poor observations.”
—Claude Bernard (1813-78) French physiologist, 1865.
MESSENGER flew 200 kilometres above Mercury’s surface on 14 January at a speed of 6 kilometres per second relative to the planet. It is the first spacecraft to visit Mercury since NASA’s Mariner 10 flew by the planet three times in 1974 and 1975. Mariner 10 was only able to photograph 45% of Mercury’s surface. MESSENGER took more than 1213 images during its flyby, covering about half of the previously unseen portion of the planet. On January 30th the first impressions of the science team were presented. As usual, more mysteries were generated than were solved. This will continue while astronomers and geologists “have an excessive faith” in their imaginary story about the birth and evolution of the planets.
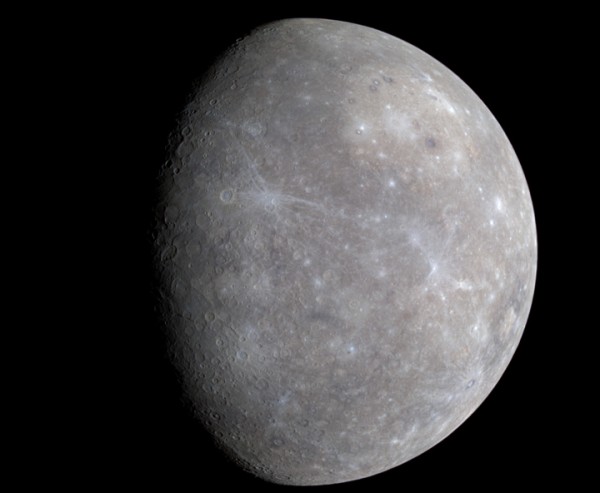
Credit: NASA/Johns Hopkins University Applied Physics Laboratory/Carnegie Institution of Washington.
From the official MESSENGER website:
“Mercury, Venus, Earth, and Mars are terrestrial (rocky) planets. Among these, Mercury is an extreme: the smallest, the densest, the one with the oldest surface, the one with the largest daily variations in surface temperature, and the least explored. Understanding this ‘end member’ among the terrestrial planets is crucial to developing a better understanding of how the planets in our solar system formed and evolved. To develop this understanding, the MESSENGER mission, spacecraft, and science instruments are focused on answering six key outstanding questions that will allow us to understand Mercury as a planet.”
Question 1: Why is Mercury so dense?
The high density of Mercury is inferred from its surface gravity, which is virtually the same as that on the larger planet, Mars. Mercury’s density implies that a metal-rich core occupies at least 60% of the planet’s mass, a figure twice as great as for Earth. The problem for theorists is that Mercury is so dense that it must contain twice as much iron relative to rock as the other inner planets.
Answer: The answer to this question was outlined in Astronomical Myths of Mercury & the Sun. The problem is that physicists have no understanding of gravity beyond the mathematics. Gravity is directly related to the mass of a body yet the relationship of mass to matter remains a mystery to philosophers of science. Physicists merely assume that gravity is directly related to the quantity of matter in a body. If that isn’t so, the quantity of matter in a body cannot be calculated from measurements of a body’s gravitational strength, and therefore its density cannot be determined. The make-up of Mercury is unknown.
The similarity in appearance of Mercury to the Moon and the tilts of their equators and orbital planes to the ecliptic, suggests they are related. It is likely therefore that they have a similar surface mineral composition. There is already evidence that the reflectance spectrum of the surface of Mercury bears a close resemblance to a laboratory spectrum of an Apollo 16 lunar highlands soil sample. And microwave spectra from the surface of Mercury resemble those from the lunar highlands. I expect therefore that measurement of the moment of inertia of Mercury will show a more homogeneous planet than one with a massive iron core. If so, that will intensify the mystery of Mercury’s magnetism (see below).
Question 2: What is the geologic history of Mercury?
From the official MESSENGER website:
”There are three major theories to explain why Mercury is so much denser and more metal-rich than Earth, Venus, and Mars. Each theory predicts a different composition for the rocks on Mercury’s surface. According to one idea, before Mercury formed, drag by solar nebular gas near the Sun mechanically sorted silicate and metal grains, with the lighter silicate particles preferentially slowed and lost to the Sun; Mercury later formed from material in this region and is consequently enriched in metal. This process doesn’t predict any change in the composition of the silicate minerals making up the rocky portion of the planet, just the relative amounts of metal and rock. In another theory, tremendous heat in the early nebula vaporized part of the outer rock layer of proto-Mercury and left the planet strongly depleted in volatile elements. This idea predicts a rock composition poor in easily evaporated elements like sodium and potassium. The third idea is that a giant impact, after proto-Mercury had formed and differentiated, stripped off the primordial crust and upper mantle. This idea predicts that the present-day surface is made of rocks highly depleted in those elements that would have been concentrated in the crust, such as aluminium and calcium.”
Answer: The three theories above demonstrate blind ‘faith’ in the idea that the planets were formed from a nebula as one ‘family,’ roughly where we see them today. There is no scientific reason to adhere to such a belief while there is an avalanche of data from space to contradict it. We do not know that Mercury has a high density. There is no need for far-fetched scenarios involving the early Sun or a colossal impact. Planet and star formation by gravitational accretion has never been observed and it cannot be shown to work in theory.
“There is a general belief that stars are forming by gravitational collapse; in spite of vigorous efforts no one has yet found any observational indication of confirmation. Thus the ‘generally accepted’ theory of stellar formation may be one of a hundred unsupported dogmas which constitute a large part of present-day astrophysics.”
—Hannes Alfvén, G. Arrhenius, Evolution of the Solar System, NASA 1976.
The alternative ELECTRIC UNIVERSE® cosmogony (theory of creation of the Solar System) is unknown to astrophysicists. It is radically different from the nebular theory of planet formation. It unites an interdisciplinary forensic investigation of the entire human record of the appearance of the sky, stretching back to Neolithic petroglyphs, with experimentally confirmed modern plasma cosmology. Because of its broad interdisciplinary base, the ELECTRIC UNIVERSE® has great explanatory and predictive power—key measures of a good theory.
There are two possible electrical origins of planets in the solar system. First, the Sun may become electrically unstable and eject sufficient matter to form a gas giant companion. Currently, astrophysicists are unable to account for powerful jets of matter seen issuing from stars and galaxies. The electrical model interprets them as electric discharge phenomena. So stars undergoing a nova outburst may give birth to gas giant planets, which explains the discovery of giant planets in very close orbits about nearby stars. It is similarly proposed that rocky planets are ‘born’ fully formed by material jetted from within a brown dwarf star or gas giant planet undergoing an electrical ‘flaring’ or ‘nova’ outburst. As British physicist Peter Warlow wrote in 1982:
“…the obvious place for a small heavy planet to form is at the core of a large gaseous planet. This is the ideal place to collect together the heavier elements and if, for the same but unknown reason that quasars eject material from their cores, the core of that large planet is also ejected, then we will have a source of Earth-like and Venus-like planets. If the lesser nova eruptions of stars are, in fact, manifestations of the same process, then we have a source of the larger Jupiter-like planets.”
— The Reversing Earth, 1982.
The second possible origin for giant planets like those in our solar system, which orbit at great distances from the Sun, is electrical capture. That would explain the great differences between Jupiter, Saturn, Uranus and Neptune. But how do we know there are any free-floating gas giants in the galaxy? By tracing galactic magnetic fields, plasma cosmologists have shown that electric current flows along the spiral arms of a galaxy. Like cosmic streetlights, all stars in interstellar space are connected to the galactic power lines (Birkeland current filaments). So instead of being dark and dead, independent giant planets shine as dim stars — red or brown dwarfs. And these dwarf stars are the most numerous in the galaxy.
The cross-section for electrical capture of a dwarf star by the Sun is huge, involving the plasma sheaths of both bodies. For example, the Sun’s plasma sheath is roughly 200 AU in diameter or .07% of the distance to the star system of Alpha Centauri. When stellar plasma sheaths touch the two stars ‘see’ each other electrically for the first time and an ‘anomalous’ acceleration toward the Sun (also experienced by charged spacecraft) takes the interloper in its grip. The electric light of the dwarf star is snuffed out and it becomes a gigantic comet, flaring and fragmenting to form new satellites, comets and ejecta rings before settling as a new gas giant into a solar orbit that provides electrical equilibrium.
In this scenario the best we can do with an obscure history of random solar system gatecrashers is to look at the planets today and try and figure out the relationships between ‘parent,’ ‘child’ and ‘foster-parent.’ Given Mercury’s odd eccentric orbit and the tendency for charge exchange to facilitate capture and circularization of planetary orbits, it seems that Mercury only lately arrived at its present location. The Sun is merely Mercury’s foster-parent. Similarly, the Earth is the Moon’s foster-parent. For to identify the parent of Mercury and the Moon we must look for family traits amongst the moons of the gas giant planets in the outer solar system. Jupiter is a prime suspect with its orbital and axial tilt being of the right order to have launched Mercury and the Moon. Both bodies would look at home among Jupiter’s Galilean satellites.
Q: Which one is the Moon? A: Second from the top. Mercury is about the same size as Callisto (bottom).
Conventionally, the geological history of Mercury is based on the study of surface features in terms of the nebular hypothesis. The heavily cratered surface of Mercury is attributed to a violent period in the development of the early solar system known as the “Late Heavy Bombardment.” This is an imaginary episode of solar system evolution. The origin of this late bombardment is unknown. It is merely required to explain craters on the Moon, Mars and Mercury. It doesn’t explain why the cratering of the Earth is so different from that on the Moon. Historically, only two mechanisms—volcanism and impact—were considered for crater formation. A century ago it was the subject of hot debate. The geologist, William Morris Davis, wrote in 1922 that “astronomers tended to explain the craters of the Moon by volcanic action, a geologic process, while geologists tended to explain them by meteoritic action, an astronomic process – each scientist evidently feeling free to take liberties with a field other than his own.” However, the ‘fact’ of impact cratering has not been observed or established by experiment. This ‘pseudo fact’ was established by consensus only after long argument between geologists and astronomers. But consensus isn’t science. The real fact is that the features of most planetary craters cannot be explained by either impact or volcanism.
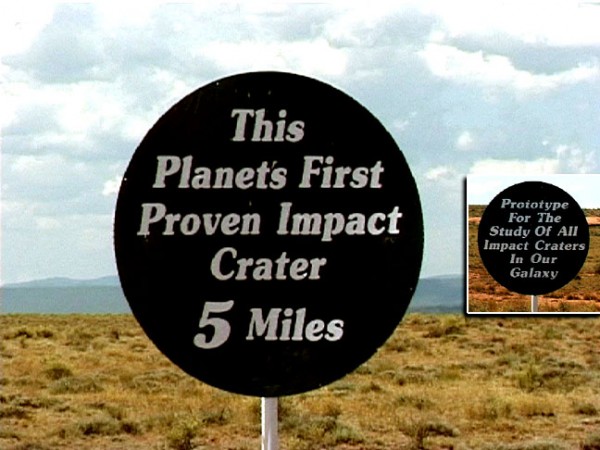
Two signs on the road to Meteor Crater, Arizona. They demonstrate the power of a belief, once it becomes established as a ‘fact.’
The Czech astronomer Zdenek Kopal was a lone voice when he scrupulously pointed out that the word ‘crater’ should be used without presupposing the mechanism of its origin. Otherwise, he warned, it could “easily render the word as much a misnomer as the Martian ‘canals’ or the lunar ‘seas.’” His warning went unheeded.
Crater circularity argues against impacts at significant angles from the vertical. So geologists assumed that the kinetic energy of the impactor is simply converted into a powerful surface explosion, like a nuclear bomb. This is now the accepted story. Gene Shoemaker in the late 20th century spent decades examining circular formations on Earth, in an effort to determine whether volcanoes or impacts formed them. His research ruled out volcanism as the cause of certain craters found on Earth. From his point of view, this left only the possibility of impact. Since then, about 160 circular formations on Earth have been identified as impact craters, although fragments of meteorites are found only around the smallest of these craters.
When the lunar samples were returned to Earth by the Apollo astronauts and up to 90% by weight was found to be shocked and welded minerals, known as breccias, all doubt about the impact origin was erased. The geologist, Robert Dietz, made the argument clear, “Barring the unlikely possibility of a natural nuclear explosion, a meteorite impact is thus the only mechanism for producing intense shock on a large scale (a lightning bolt might do so on a small scale).” Despite not knowing where the impactors came from, Kopal’s fears were realized; the word “crater” became synonymous with “impact.” Dietz deserves credit for recognizing (albeit parenthetically) that a lightning bolt could be responsible for shock and heat effects too. The mistake that he and all other geologists have made is to listen to astronomers and limit their imagination to huge impacts in the past, while dismissing huge thunderbolts.
Meanwhile, in 1965, British amateur astronomer Brian Ford showed that neither of the two main theories could account for all of the features of lunar craters and he introduced a third possibility. He noted the similarity between cratering patterns on the Moon and the microscopic patterns created by the industrial process of electrical discharge machining (EDM). Using EDM, a surface is eroded to a precise depth by myriad tiny electric discharges that form microscopic craters. Ford noticed three outstanding features of these electrical craters:
• The larger EDM craters have central peaks in about the same proportions as found on the Moon.
• The circular dish-shaped electrical craters matched the shape of the smaller lunar craters precisely.
• The larger craters often have smaller craters perched on their rims. There are no examples of a large crater intersecting a smaller crater in the same manner.
Ford also noted in his paper that the pattern of rays around the conspicuous lunar “rayed” craters is tangential to the rim of the craters. This is an impossible coincidence for impact cratering. If the craters were formed by impact, the rays would be directed radially from the center of the crater. However, this strange detail is explained electrically because the rays trace the path of electron streamers rushing from halfway around the Moon to satisfy the discharge, which is impinging inside the crater rim and not at the crater center.
Crater distribution is a puzzle too. For example, in images of any substantially cratered surface we see small craters centered precisely on the rim of a larger crater. At a 1965 NASA symposium on the nature of the lunar surface, Eugene Shoemaker remarked “there are a good many more pairs of craters, where two craters occur close together, than we have any right to expect just from random distribution.”Thomas Gold added that the two craters “are characteristically of a similar age and appearance.” That led to an ad hoc proposal that meteoroids go around in pairs. However, it is fanciful to suggest that their separation would generally allow the smaller object to arrive after the larger had formed a crater, and in such a way as to neatly target the new crater rim. We do not find a large crater that has cut into one of smaller diameter.
Difficult though it may seem, we must find a mechanism that naturally tends to form more than one crater at a time, with the smaller crater being formed after the larger crater. Also, the second crater must be formed gently so as to not disturb the first unduly. The evidence suggests that these craters are somehow ‘machined’ into the surface. There is a natural phenomenon that fits these unusual requirements – the lightning bolt. Each bolt of lightning is usually made up of more than one stroke, with the first being the most powerful. Therefore, the first stroke forms the largest crater. Any subsequent stroke will be directed at the nearest high point, usually the new rim of the first crater. These secondary craters will generally be smaller than the first. Mercury sports countless examples.
The progression of a series of electrical craters forms a crater chain. It underlines the discriminatory blindness of belief that the many neatly ‘machined’ crater chains seen throughout the solar system are attributed to chaotic impact fallout. It is impossible for low-angle impact ejecta to form neatly graded circular craters with little disturbance of one crater by its neighbor.
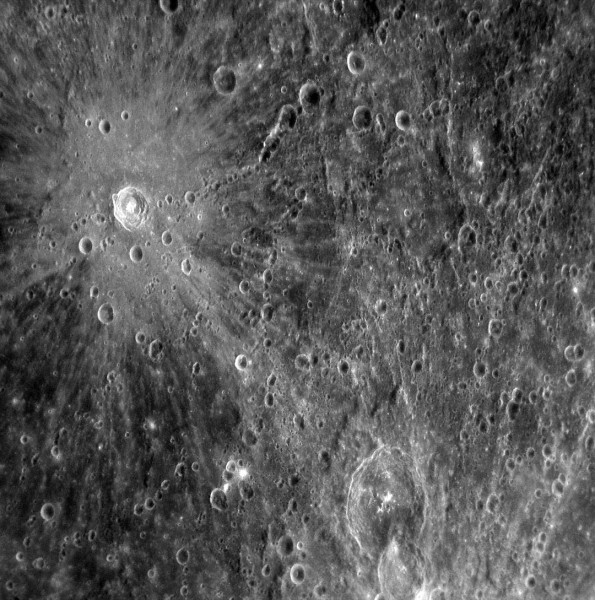
A good example of crater chains on Mercury. They cannot be explained by impacts. Credit: NASA/Johns Hopkins University Applied Physics Laboratory/Carnegie Institution of Washington.
A storm of celestial thunderbolts removes the foundation for dating a planetary surface by counting craters or measuring rock ages by using long-lived radio-isotopes. An entire hemisphere may be saturation cratered with neat, circular scars in a matter of hours. The Apollo astronauts remarked upon the fresh appearance of the lunar craters, which are supposed to be billions of years old. A short-term electrical encounter can explain the observed hemispheric dichotomies and the restricted range of crater sizes on some planets and moons.
MESSENGER finally got a look at the entire great Caloris basin on Mercury and found it to be 1,550 kilometres in diameter. Caloris is always referred to as “a giant impact structure.” But it was not formed by impact because electrical exchanges between massive celestial bodies act to prevent collision. If the sizes of the two bodies involved are markedly different, the smaller body may undergo catastrophic electrical disruption, rather like an exploding capacitor. (The effect is witnessed but unrecognized by astronomers in the form of cometary outbursts). The result may be a shower of finely divided meteoric material upon the larger body. A recent trivial example on Earth was the enigmatic Tunguska explosion. But if the Caloris basin is not due to impact, what is it?
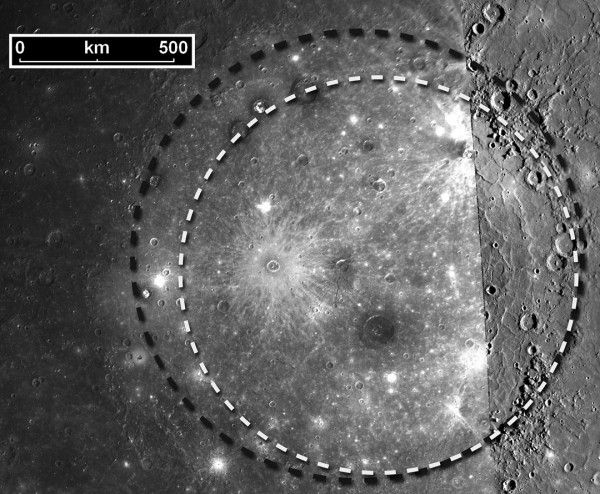
The black circle shows the size of the Caloris basin. It is larger than the estimate from the earlier Mariner image (white circle and right strip of image). The difference in lighting renders MESSENGERS more detailed image rather featureless. Credit: NASA/Johns Hopkins University Applied Physics Laboratory/Carnegie Institution of Washington.
With increasing size, craters grade into basins—circular structures with an arbitrary lower limit of about 200 km in diameter. The basins show a variety of morphologies depending on their size and degree of flooding by plains materials. The smaller basins tend to have two well-preserved rings, with the diameter of the outer ring close to twice that of the inner ring. Both rings are of relatively low relief. Outside the outer ring, radial structures dominate, consisting of hills, valleys, gouges, and strings of craters.
A feature of intense plasma discharges in vacuo is that they may take the form of a hollow relativistic electron beam. On Earth, the circular auroral curtains in the ionosphere are a result. But Mercury has no atmosphere so a similar discharge would reach the surface.
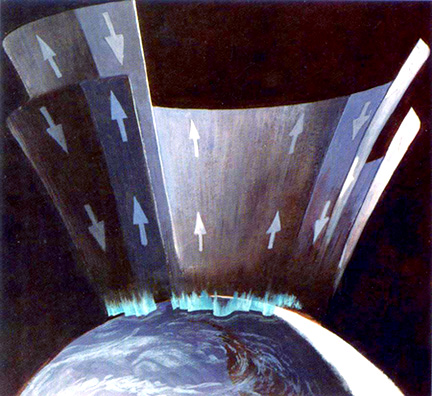
Artist’s depiction of Birkeland currents flowing in and out of the Earth’s atmosphere at high latitude. (Courtesy of S. G. Smith, Applied Physics Laboratory, The Johns Hopkins University).
These auroral currents, once the subject of intense debate, are routinely measured by today’s satellites and have total magnitudes of millions of amperes (mega-amperes). In the case of the interplanetary thunderbolt, we are talking about billions of amperes (giga-amperes). Such a powerful current will magnetically ‘pinch’ down to produce circular ringed craters and features like Caloris. Current flows radially between the current cylinders through the surface layers causing melting and etching of the crater floor or basin. So, paradoxically, a more sustained but widespread (and therefore lesser current density) discharge was probably responsible for the huge Caloris basin. The pattern of ‘fractures’ on the floor of Caloris basin is similar to the radial and concentric discharge patterns seen in the dense plasma focus device where the discharge current is forced to flow radially between two concentric conductors.
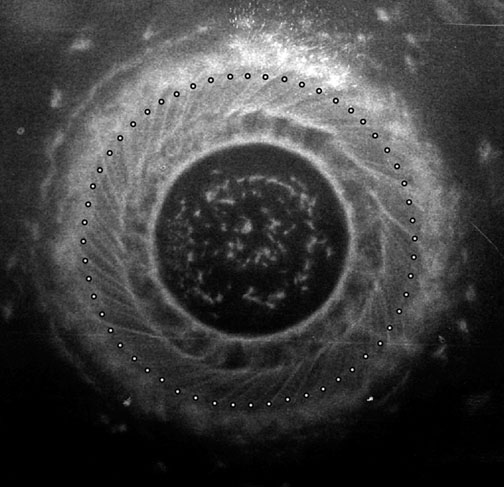
The penumbra of a dense plasma focus discharge.
It should be noted, as Tommy Gold did, that an impact causes little melting. In the brief interval of the impact the poorly conducting lunar rock cannot transfer appreciable heat. Instead, the rock simply flows until the blast over-pressure drops at which time the rock ‘freezes,’ bearing the imprint of the blast. So images of ripples frozen in the act of spreading from an object dropped into a liquid milk/cream mixture, used ad nauseum to explain the formation of ringed impact structures, is an inappropriate model. Clearly, it does not explain why two rings should be favored in the intermediate sized ‘craters.’ And central peaks are not due to ‘rebound’ from an impact. As Brian Ford noticed, they are a normal result of electric discharge machining.
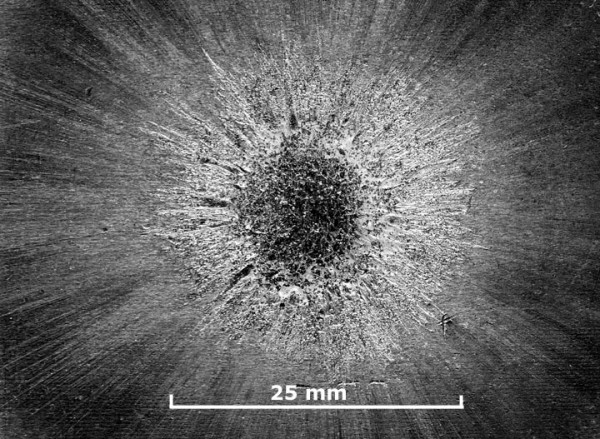
A 7 kilovolt 5 kilojoule impulsive blast on an aluminium block shows the metal frozen in a radial blast pattern that would be expected from a vertical impact of the same energy. This is the kind of pattern we should expect to see if the impact cratering theory were correct.
There are differences in arc scarring effects depending upon the polarity of the discharge. An arc to an anode (positive) surface tends to stick to one spot and cause melting and uplift with relatively subdued features. An arc to a cathode (negative) surface tends to jump about, forming many circular craters with sharp features, rim craters and chains of craters. Electrons scavenged from the surface surrounding the main crater may form ‘rays,’ and sinuous rilles or channels. The rilles often have on-channel cratering or form a crater chain that points toward the main crater. This prominent effect has given rise to the indefensible notion of secondary impact cratering due to ejecta from a large crater.
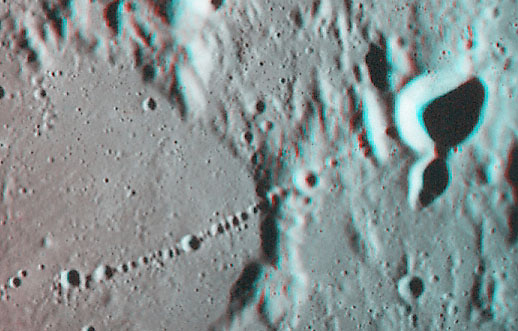
This 3D Apollo 16 image shows the well-known crater chain on the Moon called Catena Davy. The official explanation says it “..may be a chain of volcanic craters or a chain of secondary craters formed by the large Orientale impact basin located 2000 kilometers to the west. Alternatively, it may have been formed by the impact of a comet similar to Comet Shoemaker-Levy 9, which split into numerous fragments in 1992 and struck Jupiter in 1994.” Note, however, the neat circularity of all of the craters, even on the mountainsides! And Shoemaker-Levy 9 did not leave a neat line of closely spaced impact marks on Jupiter. The confusion of ideas is obvious.
The Planetary Society reports:
Louise Prockter, instrument scientist for the MESSENGER camera system, explained the science team’s struggle to interpret this feature. “What isn’t clear is the relationship of the crater to the radial trough complex. Did the crater help to form some or all of the troughs? Did it impact serendipitously right at the bull’s-eye? At this point we really don’t know. This feature is very close to the center of the Caloris basin. We have seen a number of impact basins in the solar system. We have never, ever seen a feature like this at the center of any of them. And we haven’t seen any features like this elsewhere on Mercury or on the Moon. Being scientists we have many theories as to how it formed, but at this point it’s anybody’s guess; it’s a very unexpected find.”
Answer: The Spider is unquestionably an electrical crater. Being near the center of the Caloris basin, it may have been burnt into the surface by the equivalent of a lightning ‘leader stroke,’ which sets up a conductive plasma path for a powerful return stroke. That would account for the crater being slightly off-center in the Caloris basin. The radial channels or ‘rilles’ are diagnostic of material being blasted aside by surface lightning as electrons are stripped from atoms and follow the electric field toward a high central point where they launch into space, forming a crater. It is obvious that the largest rille, entering the picture bottom center, was responsible for initiating the 40 km electrical crater, with its steep walls, flat floor and central peak. None of these features are explained by an impact. The crater walls also hint at the polygonal ‘diocotron instability’ pattern of a powerful particle beam.
Planetary scientists have missed a new, low cost field of research into the most powerful sculpting force of planetary surfaces—the electric arc. Meanwhile, plasma physicists and electrical engineers under the auspices of the largest professional body in the world, the Institute of Electrical and Electronic Engineers (IEEE), published a paper last year on the production of Martian ‘blueberries’ by an electric arc striking an analog of Martian soil. Specialization and institutionalisation of science takes its toll of progress. Astrophysicists and planetary scientists don’t heed the IEEE plasma science publications. They operate in an imaginary ‘parallel’ universe detached from the obvious electrical nature of our real universe.
Question 3: What is the nature of Mercury’s magnetic field?
Answer: This was explained in the 14th January news item referred to earlier. In Mercury’s case, its strong gravitational field for its size indicates a high level of internal electrical polarization. That means a high surface charge. So Mercury’s slowly rotating charge will produce a weak dipolar magnetic field.
Question 4: What is the structure of Mercury’s core?
Answer: This is an interesting test of the ELECTRIC UNIVERSE® model which has no need of an immense core of liquid iron. Measuring Mercury’s moment of inertia provides one test. Currently it has not been measured, but conventional interior models constrain it to the range from 0.325 to 0.380, where the smallest value corresponds to the most centrally condensed configuration. When MESSENGER goes into orbit about the planet and measures the gravitational field globally we will learn if it is a more homogeneous sphere or not and whether it has a liquid or solid core. I expect Mercury’s moment of inertia to be closer to 0.4, in other words closer to a homogeneous sphere and rather like the Moon. Also, by analogy with the Moon, it is probable that Mercury’s core is solid.
Question 5: What are the unusual materials at Mercury’s poles?
Answer: Radar imaging of Mercury from the Earth in the early 1990s found anomalous radar-bright returns from the Martian poles. They have raised the possibility that water-ice survives in some of the polar craters where the Sun never shines. Discovery magazine also reports that, “The first rock from the sun has a glowing dragon tail of sodium atoms that is more than seven times longer than ever suspected.” These findings fit a planet that is strongly transacting electrically with the solar plasma. The electric current will follow Mercury’s magnetic field. We may expect therefore that plasma discharge effects will occur most strongly along sharp crater walls near the poles. Such vertical plasma discharge curtains, similar to those observed at Io by the Galileo orbiter, will return a strong radar echo if sufficiently dense. (Plasma can reflect a radar signal like a mirror). It seems less likely that matter suspended in water ice on the floor of a crater would return an appreciable signal at such low grazing angles. The estimated extent of the radar reflective region of 350km is of the right order for an auroral-type discharge.
Electrical spark machining of Mercury’s surface provides the source and energies of sodium, potassium and other ions that have been detected in Mercury’s tenuous atmosphere (exosphere) and cometary plasma sheath. A concentration of potassium ions above the Caloris basin suggests a magnetic anomaly and/or ion implantation remaining from the cosmic thunderbolt that struck there.
Question 6: What volatiles are important at Mercury?
From the official MESSENGER website:
”Six elements are known to exist in Mercury’s exosphere: (1) hydrogen, (2) helium, (3) oxygen, (4) sodium, (5) potassium, and (6) calcium. The observed exosphere is not stable on timescales comparable to the age of Mercury, and so there must be sources for each of these elements. Hydrogen and helium are present in high abundances in the solar wind, the stream of hot, ionized gas emitted by the Sun. The other elements are likely from material impacting Mercury, such as micrometeoroids or comets, or directly from Mercury’s surface rocks. Several different processes may have put these elements into the exosphere, and each process yields a different mix of the elements: vaporization of rocks by impacts, evaporation of elements from the rocks due to sunlight, sputtering by solar wind ions, or diffusion from the planet’s interior. Variability of the composition of Mercury’s exosphere has been observed, suggesting the interaction of several of these processes.”
Answer: The most important process missing from the list is that of electric discharge machining (EDM) of Mercury’s surface. The problem of the astrophysics mind set can be seen in the language used, “the stream of hot, ionized gas emitted by the Sun” is better understood by plasma physicists as an equatorial solar current sheet rather than a hot wind. Also the exosphere has not existed for the “age of Mercury” since Mercury has not been in its present orbit for as long as astronomers believe. So we can expect more surprises when MESSENGER goes into orbit about the planet — as usual.
Messenger will fly by Mercury twice more, on 6 October 2008 and 29 September 2009. Mercury’s gravity slows Messenger down during each flyby, making it easier for the planet to eventually capture the spacecraft into orbit on 18 March 2011. The spacecraft is designed to last at least one year in orbit around Mercury.
Wal Thornhill