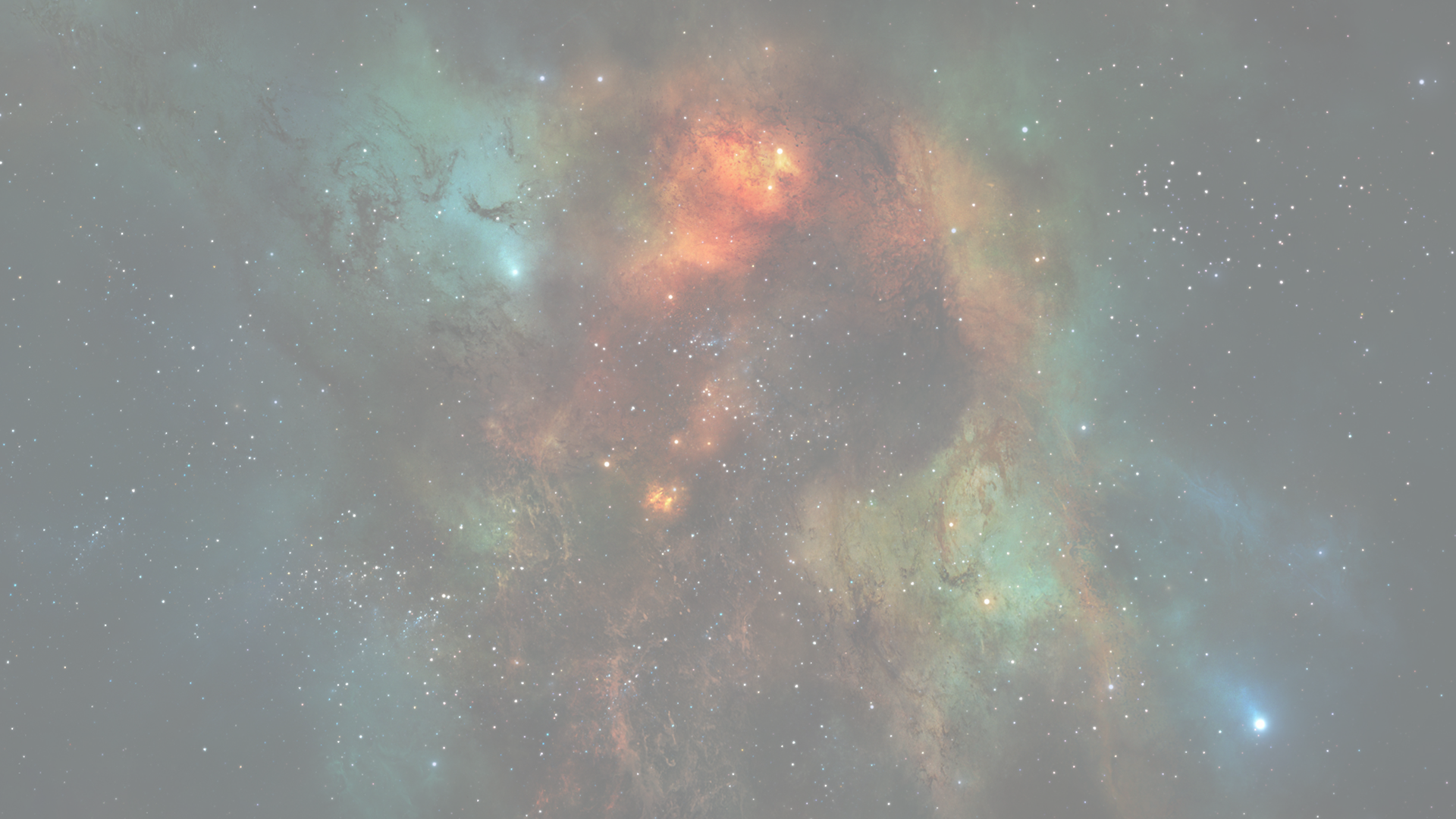
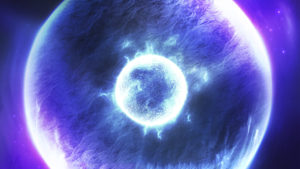
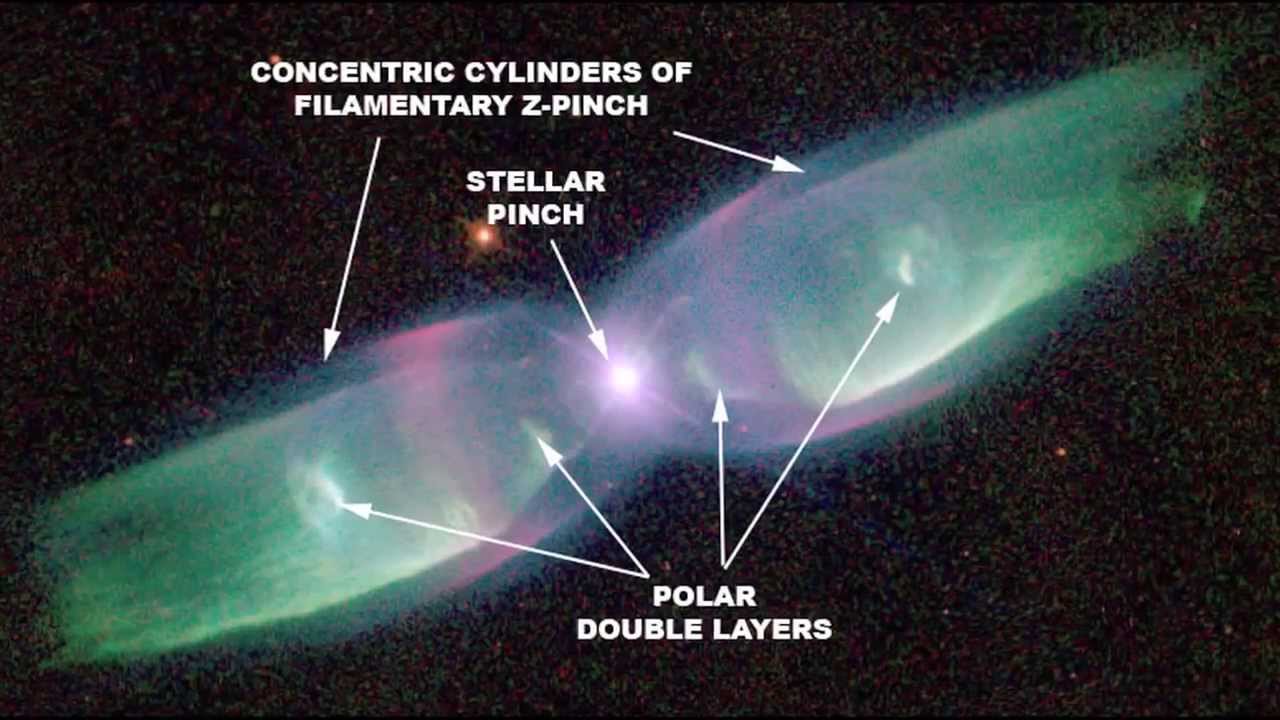
Plasma Cosmology
Theories of the Universe
We still have a lot of material to cover in this section, and I want to give you a brief overview of an interesting alternative to conventional cosmology. So I'm going summarize for you these two challenges to the big bang and include a third problem as well. The test of any scientific theory is based upon the relationship between its predictions and observations. Let's see how well the big bang has done:
Universal Constants
Many cosmologists think that nearly 99 percent of the universe is unobservable and made of dark matter. The universe we do see, the stars, galaxies, and literally everything else, only constitutes about 1 or 2 percent of the total amount of matter in the universe. The rest is some strange and unknown form of matter, particles that are necessary for the big bang theory to work. Theorists realized that there is just too little matter in the universe for the gravitational forces to have created the universe in the form that it's in today. So something has to create the needed gravity, hence the theory of dark matter. This idea was introduced about 20 years ago and has since become a fundamental part of the big bang cosmology.
Plasma is regarded as a fourth phase of matter, the other three being solid, liquid, and gas. It is a hot state of matter in which electrons have been stripped from atoms to leave positively charged ions, which mingle freely with the electrons. The Northern lights are a naturally occurring form of plasma, as is St. Elmo's fire. You've probably seen the “Eye of the Storm” or similar plasma balls in stores. They're those really cool objects that have the tiny electrical storms inside the glass spheres. When you bring your hand in contact with the glass surface, the bolts of plasma electricity inside react to ions surrounding your hand.
- It predicts that there should be no object older than 20 billion years and larger than 150 million light years across. And as we've discussed, that's certainly not the case.
- It predicts that the universe, on the large scale that it exists, should be smooth and homogeneous. It's not—it's clumpy!
- The third problem has to do with the strongest evidence in support of it, cosmic microwave background radiation. In order for the universe to produce the galaxies we see around us, the fluctuations found in the background radiation indicates that there must be a hundred times more dark matter than visible matter. But there is no experimental or observable evidence that dark matter exists. It's a theory to make the big bang work. So if there is no dark matter, the theory predicts that we can't have galaxies, but we live in one—the Milky Way.
So while the big bang predicts the things in the preceding list, observations have shown them to be incorrect. However, this is the accepted theory for now, and many scientists assume that it's right. To abandon it would not be easy. Few theories in science are ever left behind when there is no alternative in sight. So what are we left with? Well, there is a new alternative on the horizon. It's called plasma cosmology. Here's a basic idea of what it's about.
The advocates of plasma cosmology believe that the evolution of the universe in the past must be explained in terms of the processes occurring in the universe today. In other words, events that occur in the depths of space can be explained in terms of phenomena studied in the laboratories on earth. This approach rules out the concepts of a universe that began out of nothing, somewhere in time, like the big bang. We can't recreate the initial conditions of the big bang in laboratories. The closest we can get is in the particles created in accelerators. Plasma cosmology supports the idea that because we see an evolving universe that is constantly changing, this universe has always existed and has always evolved, and will continue to exist and evolve for eternity.
Another aspect of this new theory is that, while the big bang sees the universe in terms of gravity alone, the plasma universe is formed and controlled by electricity and magnetism, not just gravitation. With the introduction of electromagnetism the “clumpiness” of the universe and the fluctuations in microwave background radiation can be easily accounted for. Even the expansion of the universe can be explained by the electromagnetic interaction of matter and antimatter.
Cosmonotes
Since all that is being provided for you is a simple summary and basic explanation of plasma cosmology I would recommend that you check out the list of recommended reading in this area in the Appendix B, “Suggested Reading List.” There is a lot more to this theory than I can elaborate on in the space of a few pages, so if you're interested in finding out more about these new ideas, I suggest you look into some of the books I've recommended. There is still very little support for this theory because the big bang is the one that many believe is the correct interpretation of the origin of the universe, and to question the validity of this theory is not on the minds of many of today's cosmologists.
And while electromagnetism forms the basis for plasma cosmology, it is also the basis for our technological society that surrounds us today. Plasma technology has stimulated research for better computer screens, how radio and radar transmission can be increased, and may be the answer to developing the long-sought-after genie in the bottle: fusion energy. So in the long run it holds the possibility of not only providing a better description of the origin and structure of the universe, but it can also lead to a whole new area of advanced technology. I'll be discussing some material on the Eastern traditions and their approach to cosmology. There are some interesting correlations between their understanding of the universe and the ideas behind plasma cosmology.
Anthony Peratt
Plasma Cosmology
Anthony L. Peratt, Los Alamos National Laborat'ory
ASIMOV’S “Nightfall” tells the of a civilization on a planet with
six suns, where night comes only once every 2,049 years. Scholars of that world have uncovered traces of at least nine previous cultures, all of which reached a height comparable to their own and then vanished suddenly.
Because of their viewing handicap, those scientists’ cosmology is faulty. At their most creative, they can only imagine that their universe consists of perhaps a few dozen “stars” — mysterious lights that eccentric cultists are forever talking about. When night does fall and myriad stars shine forth, their cosmology, and indeed the philosophical basis of their society, crumbles.
Until recently our own view of the universe also was handicapped, limited to information derived from the narrow range of wavelengths that make up visible light. About the middle of this century our spectral window expanded to include infrared and radio radiation. Then, begin- ning in the 1960’s, space research opened up the ultraviolet, X-ray, and gamma-ray regions of the spectrum as well. Today only very long-wavelength celestial radio waves remain unknown to us. They are blocked by the magnetosphere, a protec- tive cocoon that envelops Earth.
Most of the radiation in the spectrum comes from something called plasma. This is a fourth state of matter, different from a solid, liquid, or gas, but most closely resembling the last. However, unlike a gas, whose component atoms or molecules are electrically neutral, a plasma is made up of charged particles.
A plasma can form when a gas is heated to such a high temperature that collisions ionize it by tearing electrons from atoms. The result is a cloud of free, negatively charged electrons and positively charged ions, atomic nuclei with one or more of their attendant electrons missing.
The term plasma also includes ionized
The plasma universe may be eternal and infinite, directly contradicting the Big Bang model. In this nonstandard picture, swirling streams of electrons and ions form filaments that span vast regions of space. Where pairs of these spaghettilike structures interact, the particles gain kinetic energy and at narrow “pinch” regions produce the entire range of galaxy types as well as the full spectrum of cosmic electromagnetic radiation. Thus galaxies must lie along filaments, much as they are observed to do on a large scale. The bulk of the filaments are invisible from a distance, much like the related Birkeland currents that circle Earth but are unobservable from its surface.
gas at a relatively low temperature, where only some of the atoms or molecules have lost electrons. This state of matter even exists inside a metal at room temperature. In this case the conducting electrons in the solid are free to wander through the rigid crystal lattice of metallic ions.
Because of its free electrons, a plasma is a good conductor of electricity, much better than copper, silver, or gold. Light- ning offers one of the most dramatic
manifestations of this property. As a thunderstorm develops, negative charges accumulate along the cloud base, causing positive charges to build up on the ground below. The resulting electrical field be- tween the two concentrations becomes so strong that it ionizes the air. This creates a conducting path of free electrons and ions
— a plasma — through which the light- ning discharges.
A young engineer working for the Gen-
eral Electric Company gave plasma its
name. In 1923 Irving Langmuir, who went on to win the Nobel prize in chemistry, was fascinated with the effect of electrical discharges on gases. He borrowed the term plasma from medicine because it fitted the unstable, almost lifelike behav- ior of the ionized material with which he experimented.
While all matter is subject to gravita- tional forces, the negatively charged elec-
!asma also react to electric and magnetic
›rces that are 10" times as strong. Be- ruse of these additional interactions, plas- mas display structures and motions that e far more complex than those found in
- utra1 solids, liquids, or gases. Langmuir as among the first to note the separation I highly conducting plasma into charged- Article sheaths or cellular like walls. This ructure appears wherever samples with ifferent densities, temperatures, or mag-
- tic-field strengths come into contact.
Like flashes of lightning, terrestrial plas- mas are by and large transient. Even in a con or fluorescent bulb, the mixture of ee electrons and ions remains only as ing as the power is turned on. Extrater-
:strial plasmas are much more long-lived, ut until recently only a handful of scien- sts had speculated about the universal ttent and character of such matter. Yet lmost all of the observable universe is lasma. Stars, for example, are gravita- onally bound plasmas, while all of inter- ellar and intergalactic space is a plasma.
Supercomputer simulations of interactions between a pair of galaxy-size plasma filaments can reproduce the shapes of cosmic radio sources. The brightness maps of actual double radio galaxies in tbe top row show bewildering variety. Those at bottom are all from different stages of one simulation. The left figure corresponds to some 20 million years after the interaction begins; time increases toward the right plot, which depicts the simulated interaction some 40 million years later. These calculations suggest that apparently unrelated radio galaxies may be part of the same family, but at different stages of development.
THE PLASMA UNIVERSE
Wherever plasmas exist, they produce rodigious amounts of electromagnetic ra- iation. In particular, X- and gamma rays om beyond the solar system are likely roduced by free electrons with energies arresponding to temperatures of more ian I million degrees — the realm of hot, iagnetized plasmas. We call the overall icture obtained from these energetic emis- ons the plasma universe.
Hot plasmas also emit radiation of lower energy, such as visible and radio waves (we can both see lightning and hear it on a receiver). However, the emission does not always have a thermal origin. For example, unknowing humans have viewed synchrotron radiation (from elec- trons spiraling at nearly the speed of light in a magnetic field) from the Crab nebula for centuries.
Synchrotron radiation is named after the particle accelerators developed in the
1930’s and 1940's to produce high-energy electrons. In 1950 Hannes Alfvén, Nikolai Herlofson, and Karl Kiepenheuer brought this form of plasma radiation to astrono- mers’ attention. Alfvén, who later won a Nobel prize in physics for his solar stud- ies, proposed that streams of electrons move at nearly the speed of light along magnetic-field lines not only in Earth's magnetosphere and above the Sun, but also throughout the cosmos. If so, sheets and ropes of electric current should criss- cross the universe in ever-increasing sizes. These currents, Alfvén thought, should give the universe a cellular and filamen-
tary structure.
Astronomers accepted Alfvén's notion of widespread synchrotron radiation but refused to believe that electric currents give rise to the large-scale structure of the universe. In those days it was standard cosmological lore that the universe be- came smoother and smoother on larger and larger scales. Huge filaments, sheets, and walls of galaxies were unknown.
Modern plasma cosmologists have been heavily influenced by the earlier research of Norwegian scientist Kristian Birkeland (S&T: May, 1985, page 389). At the turn of the century he suggested that electrical currents due to “corpuscular rays” (plasma) from the Sun caused the aurora borealis. Such currents were considered impossible until they were discovered by an artificial satellite in 1974. Enormous
Complex forces between two Birkeland currents, which are electric currents aligned along
magnetic-field lines created by electrons moving in helical orbiLs around and along the
field lines. These currents have parallel components that exert a long-range attractive and circular components that provide short-range repulsion. Let: The geometry used
computer simulations. If the electrons are moving near the speed of light tbey emit synchrotron radiation beamed along their magnetic-field line, so the emission nearly mirrors the magnetic-field pattern. flight: How the forces due to the Birkeland current components vary with separation, along with the behavior of the combined (net) force.
Bir keland currents connecting Jupiter and its moon Io were recorded by the Voyager pacecraft in 1979.
In 1984 Farhad Yusef-Zadeh, Don Chance, and Mark Morris found an exam- ple of Birkeland currents on a galactic scale. Working with the Very Large Array
Fahril nrv. 1992. ?k v k Telescone 137
These images from a supercomputer simulation trace the development of spiral structure in two interacting plasma blobs over a span of nearly I billion years. At the start of tbe interaction at upper left the filaments are 260,000 light-years apart; all 10 panels are reproduced at the same scale. Simulations such as this can reproduce the full range of observed spiral-galaxy types using electromagnetic processes rather than gravitational ones. Unless otherwise noted, all illustrations are courtesy the author.
The Wolf Effect and Galaxy Redshifts
Spectral lines can be redshifted toward longer wavelengths or blueshifted toward shorter ones. The Doppler effect ex- plains how these shifts occur because of relative motions of the source and the observer along the line of sight. Ap- proach causes a blueward shift and reces- sion a redward one.
Scientists have long believed that only the Doppler effect or gravity as de- scribed by Einstein could account for wavelength shifts in the spectrum of light as it travels through space. Where neither factor applies, scientists have always assumed spectral invariance
the spectrum remains the same no matter how far the light travels. This is the case
Shifts of Spectral Lines
WAVELENGTH (angstroms)
with ordinary sources — called “Lamber- tian” after Johann Heinrich Lambert such as the blackbody radiation from stellar surfaces.
In the past few years, however, experi- ments have shown that there is a third way to shift spectral lines. This mecha- nism involves non-Lambertian sources
Left: Due to the Wolf effect beamed emission from two separate sources can interact
and shift the wavelengths of lines in their spectra. The change can be redward or blueward by different amounts depending on the observer’s point of view, but from “head-on” the shift is to the red. This is a distinctly different process than the more familiar Doppler effect. Right: Here the two mechanisms are compared for the case of oxygen lines with a redshift of 0.07. However, the light sources involved in the Wolf effect are stations , not fleeing the observer at 84 kilometers per second.
that emit beamed energy, such as lasers and devices producing synchrotron light. The discoverer of the new effect is physicist Emil Wolf, who, along with Max Born, wrote the definitive textbook Principles of Optics.
A mechanical analog to Wolf’s discov- ery is a pair of tuning forks with nearly identical resonant frequencies (pitches). If these forks are connected together mechanically by, say, a sounding board, the coupling is strong and the resonant frequencies tend to get “dragged down” qto lower ones.qqIn other swords the
wavelength is lengthened, or redshifted.
This phenomenon has been verified ex- perimentally with light waves and for sound waves from coupled speakers.
The actual frequency shift due to the Wolf effect depends on the geometry. As the illustration above shows, whether an observer sees a redshift or a blueshift depends on his or her location with respect to the source.
This mechanism can be extended from the case of two radiating point sources to that of a whole collection of such objects, for example a plasma cloud.
Wolf and his colleagues have shown that
such a cloud can produce shifts that closely mimic the Doppler effect. The figure above shows an example.
Thus the assumption that quasars beamed electromagnetic radiators with large redshifts — are part of the “Hub- ble flow” of an expanding universe could be wrong. Whether this also ap- plies to normal galaxies remains unclear. This situation, coupled with the question of the origin of the cosmic background radiation, raises the possibility that there is really no need for the Big Bang.
Filamentation — the transformation of energetic, high-temperature material into current-carrying bundles — is characteristic of plasma at any scale. Lej?: In the laboratory filaments are produced when a pulse-power generator delivers 10 trillion watts to a plasma only a few centimeters long, heating it to a temperature of 8,000,000° Kelvin. Center: Similar structure is seen in solar prominences, but in this case the lengths are measured in hundreds of thousands of kilometers. Photograph by Benny Sundstriim. flights Long, thin structures near the center of the Milky Way stretch out over roughly 120 light-years. Courtesy Farhad Yusef-Zadeh and Mark Morris. Another jump to a scale a few million times larger would bring us to the size of filaments that plasma cosmology needs to form galaxies. Thus the recent discovery of vast filaments and sheats of galaxies spanning hundreds of millions of light-years is good news for plasma cosmology. Standard cosmology assumes that the universe becomes smooth at very large scales.
radio telescope, they discovered an arc of radio emission some 120 light-years long near the center of the Milky Way. The structure is made up of narrow filaments typically 3 light-years wide and running the full length of the arc (see the image above, right). The strength of the associ- ated magnetic field is 100 times greater than previously thought possible on such a large scale, but the field is nearly identical in geometry and strength with simulations of Birkeland currents in stud- ies of galaxy formation (S&T: August, 1984, page 118).
SUPERCOMPUTING THE COSMOS
The set of equations describing how a filamentary, electrically conducting, mag- netized plasma evolves is a mathemati- cian’s nightmare! Because of this complex- ity, effective solutions had to wait for the advent of supercomputers.
Plasma theorists often use a method called particle simulation. Some tens of millions of “particles” are used to repre-
sent, say, a galaxy. But since a system similar to the Milky Way may contain 1065
free electrons and ions, each particle in the simulation actually represents a cloud of real ones. These “superparticles” are assumed to be in a magnetic field similar to that between the planets in the solar system, but much larger in size. The computer then calculates how the particles move according to the laws of electromag- netism.
The simplest simulation, whose geome- try is pictured at the bottom of page 137, traces the interaction of two Birkeland filaments made up of fast-moving elec- trons (because of their greater mass, posi- tively charged ions move more slowly and are usually ignored). No matter how many
When two magnetized plasma filaments of galactic dimensions interact, they emit an early burst of synchrotron radiation that lasts 4 million years or so.
filaments are present, the two closest to each other will always interact most strongly, because the net force between two like currents falls off in direct propor- tion to the distance between them (see the graph on page 137). This so-called Am- pere’s-law force is stronger and has a longer range than gravity, which falls off as the square of the distance.
Because electrons spiral around mag- netic-field lines, each filament has a circu- lar current component. Tvo such compo- nents repel each other and in so doing give off energy in the form of synchrotron radiation like the example illustrated above. In a typical case about 2 x 10" joules are released over an interval of some 4 million years (1 joule will raise an apple 1 meter off the ground). Dividing the energy by the duration gives a radiated
power of 103 watts. Interestingly, this is
close to the output of a strong extragalac- tic radio source like Cygnus A.
The two-current simulation was one of
the first large-scale plasma calculations. Today’s supercomputer networks are nearly 100 times more powerful than those of just a few years ago, and simulations can now involve as many as 50 million particles. The calculations provide infor- mation not only on sources’ power levels and shapes but also on their polarizations. All of these properties can be compared with results from radio telescopes.
One result •of this improved perform- ance is the ability to sort out the evolution of “double” radio sources that until now seemed unrelated. The top diagram on page 137 suggests that double radio galax- ies evolve from filamentary plasma, an- nouncing their birth through a double- beam pattern of radiation that they retain through the era of synchrotron radiation.
The radiation patterns grow more com- plex as they fade. The plasma does not disappear, however, and the illustrations at the top of the facing page show how double radio galaxies and quasars might change first into peculiar and Seyfert galaxies, then into normal and barred spirals. Filamentary plasma on supergalac- tic scales can produce a wide variety of galaxy shapes.
Calculations are now good enough that we can compare their detailed predictions with observations of how a galaxy’s rota- tional velocity varies with distance mom its center (see the illustrations on the next page). Simulations involving plasma can match the data well and do not require a large amount of “dark matter” (whatever that is) to do so.
COSMIC BACKGROUND
In one view, the radio sky is peppered with sources that chance to beam their energy toward Earth. If so, what happens
100 50 0 50
PADIUS (thousands of light-hears)
|
to the energy haphazardly radiated in
other directions?
Plasma cosmologists William Peter and Eric Lerner asked just that question. The two were intrigued by the parallels be- tween the cosmic microwave background radiation and the radiation produced by very energetic plasma generators in the laboratory.
In both cases the spectrum is that of a blackbody, a perfect absorber and emitter of energy. However, this is so in the laboratory version only because filamen- tary insulation is placed around the genera- tor to protect the environment. Could gigantic filaments of cosmic plasma act like that shielding and give the “wasted” radiation a blackbody spectrum that would fill the sky? Indeed they could.
Then what would the energy density be? The answer is a minuscule 5 x 10 " joule per cubic meter. Interestingly, this corre- sponds to a blackbody temperature of 2.87° Kelvin, in close agreement with the 2.73° K measured by the Cosmic Back- ground Explorer satellite.
MORE ANSWERS FROM PLASMAS?
Except for weakly ionized gases, all plasma is cellular, having regions with different properties separated by transi- tion zones. Does this honeycomb structure occur at increasingly larger scales without
100
f10f T tire tin:Pervert v:iiaiigc: of rotational velocity with radius in a spiral galaxy’s disk is compared with the
, results of a supercomputer simulation of a spinning object composed of two interacting plasma fila- ments. NGC 2998 is a 13th magnitude spiral in
Ursa Major. The mass used in the supercomputer cal- culations was typical of the Db8errnbfe mass of a galaxy
— its luminous stars and gas. The plasma universe needs no dark matter to reproduce such rotation curves because the electro- magnetic forces acting on plasma are so much stronger than gravity.
llllll I ' OUW icii gc cciii i ii‹iiiicii cc i oaiiJ gp We observe tiny plasma filaments in the
laboratory and ever-larger examples in the aurora, solar coronal loops, the galactic center, and perhaps the lobes of double radio galaxies and even clusters of galax- ies. Could there be even bigger examples containing superclusters of galaxies or even greater aggregations? If so, enor- mous cell walls and filaments might ex- plain the surprisingly nonuniform, very- large-scale structure of the universe.
Plasma cosmology offers other interest- ing alternative views of the universe. One example, described in the box below, involves Olbers’ paradox. Another, sum- marized in the box on page 138, explains the redshifts of galaxies without resorting to cosmic expansion, thus getting rid of the need for the Big Bang.
The plasma vision of the universe is at odds with how most cosmologists have viewed our universe for several genera- tions. It probably will be proved or dis- proved in the coming century. Either way, we are in for some exciting and interesting times now that we have reached “nightfall” and can explore the uni-
verse at almost all wavelengths.
Anthony Peratt is an associate editor of the Institute of Electrical and Electronics Engi- neers’ journal Transactions on Plasma Science and author of Physics of the Plasma Universe (Springer- Verlag, 1991).