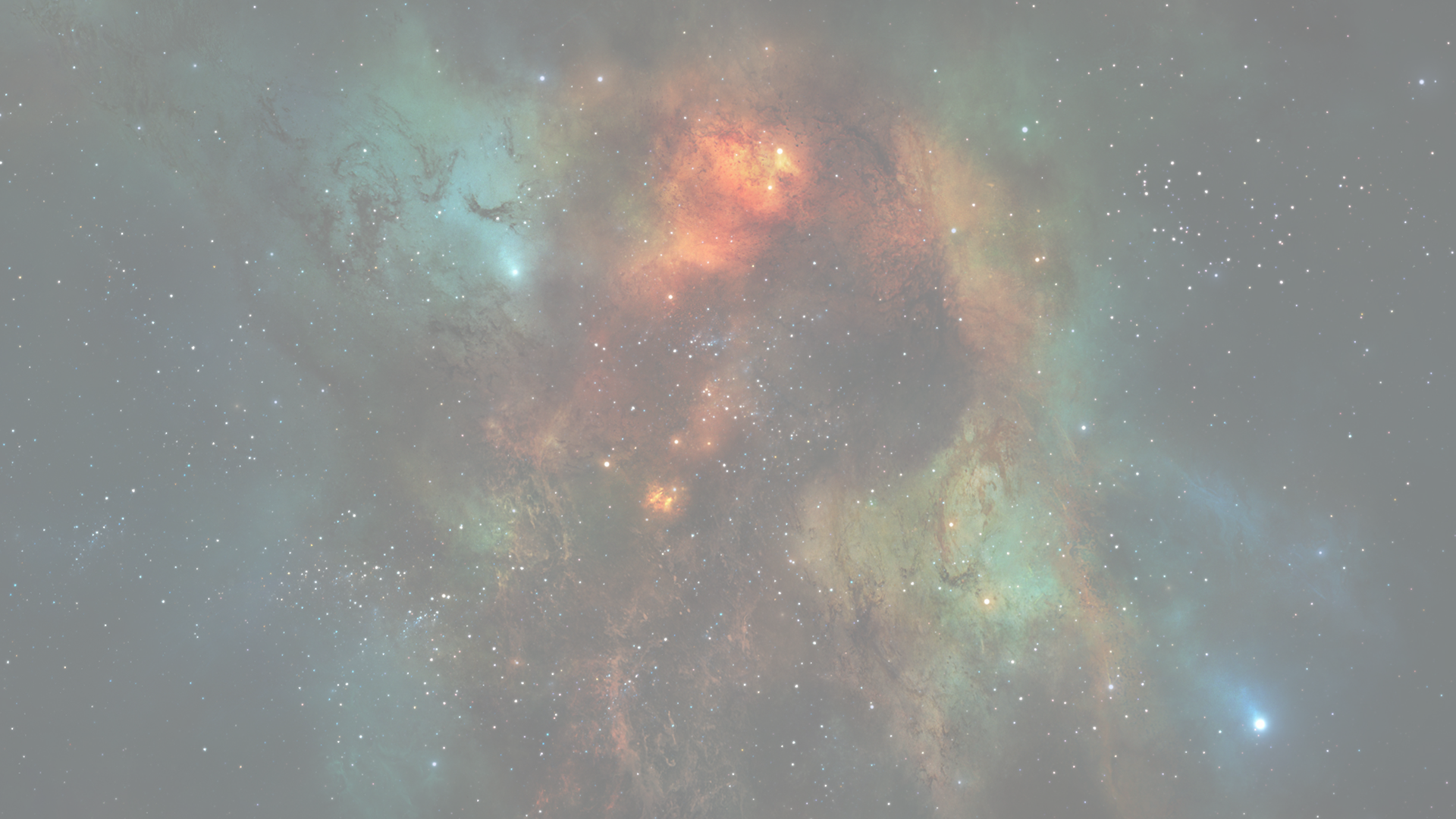
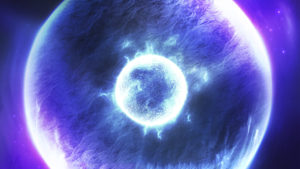
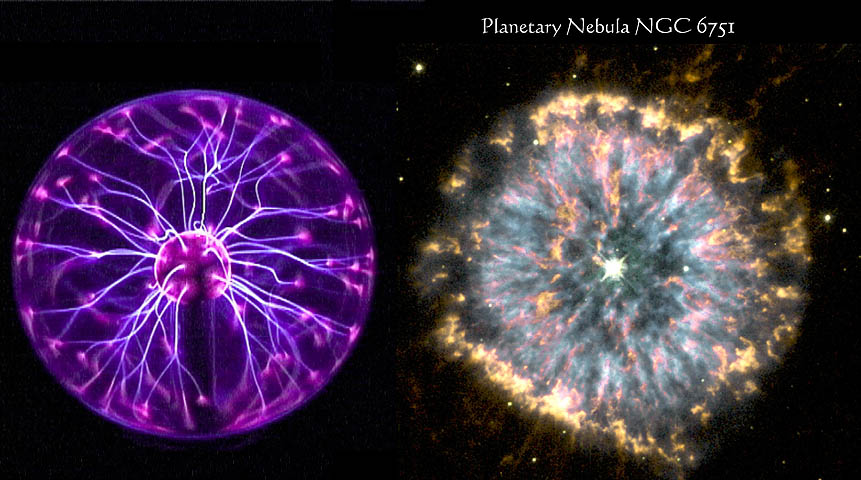
Plasma & Electricity in Space
“Not only is the universe permeated by plasmas and plasma currents, but its dynamics are dominated by electromagnetic, not gravitational, forces.”42
Understanding the ‘States of Matter’
How did astrophysicists, despite the many signs of electrical activity in the cosmos, justify their notion of an electrically sterile universe? It comes down to a failure of understanding. Experts in the behavior of gravitational systems did not understand the unique properties of plasma or the implications of a plasma universe.
A key variable affecting the physical states of matter is temperature. In solids, thermal energy is very low. The atoms are rigidly bonded to each other. As the temperature rises, the molecular and atomic bonds are loosened, allowing the solid to become a liquid. Gases form at higher temperatures, as the bonds are loosened still more, allowing the atoms or molecules to
move freely and to achieve a mechanical equilibrium within the confines of their environment.
At still higher temperatures the atoms themselves can begin to break apart in a process called ionization. Here, the electrons, or negative components, separate from the atom, leaving the remainder of the atom, the
ion, positively charged. This is a plasma. Plasma is a collection of positively charged ions and negatively charged electrons, together with the remaining non-ionized or neutral atoms or molecules.43
Perhaps the most distinctive and significant characteristic of plasma is that, unlike gases, it is an excellent conductor of electricity
—far better than copper. As a moving conductor in space, it develops electric currents and magnetic fields of its own in response to an ambient magnetic field. The result is the complex behavior that is familiar to plasma physicists.
A well-known example of plasma is a glowing neon light. The auroras of the polar skies and the atmospheric trails of meteors are also plasmas excited to a glow, as are the comas and ion tails of comets.44
In the ‘ionized’ state of matter, some or all of the discrete charged particles that make up atoms (LEFT) are dissociated (RIGHT) into separate electrons (blue) and positive ions (red) that are free to move—creating a highly conductive plasma.
42 A. L. Peratt, “Not With A Bang,” THE SCIENCES Jan/Feb 1990.
43 Of course, in deep space, far from any star, it is high-energy UV and X-ray radia- tion rather than heat that ionizes the sparse atoms.
44 The flyby of comet Borrelly by Deep Space 1 in the Fall of 2001 found plasma den- sities hundreds of times higher than expected.
Above is a laboratory discharge tube with a magnet held to the glass. The response of the plasma can be seen as the charged particles are influenced by the magnetic field.
Credit: Paul Doherty, The Exploratorium.
A novelty plasma ball demonstrates some important features of an electric discharge in a plasma. Most notable are the thin blue filaments of electric current. The filaments collect current from the large surface of the outer sphere and combine with each other to form thicker filaments on their way to the small central electrode. Even such simple objects as this can help us to understand plasma activity in space, from the multitude of electric phenomena on the surface of the Sun to the scarring patterns on neighboring planets.
Image: W. Thornhill
When plasma glows it is because the energy that produces the plasma may also excite the electrons of non-ionized atoms to higher levels. As these ‘excited’ electrons drop back they emit light. Additionally, electrons may emit light when they recombine with positive ions.
The Earth’s ionosphere is a weakly ionized plasma that does not normally glow. And the free electrons in metals should be considered as plasma too; they are the reason that metals are good conductors.
The boundaries of ‘magnetospheres’ of the Earth and of other planets are invisible plasma sheaths that define the limits of the electrical influence of the enclosed planets.
Since the excited particles are widely separated in interplanetary space, these plasma sheaths do not normally give rise to a visible glow. Nor is the solar wind visible, though it is a stream of plasma (see information panel p. 96). All of interstellar space is filled with plasma, and our Sun and all of the stars in the sky are considered to be balls of plasma. And, as argued in the last chapter, galactic formations are plasma structures as well.
One very familiar form of plasma is the lightning stroke. A terrestrial bolt of lightning consists of narrow channels of atmospheric atoms and molecules, of which approximately 20 percent are ionized. Lightning streamers are channels of plasma. But as we shall observe in these pages, the terrestrial lightning bolt has many counterparts in the near-vacuum of space, most of them yet to be acknowledged as such. Moreover, to recognize the forms of cosmic ‘lightning’ is to go a long way toward resolving the ancient mysteries of the ‘divine thunderbolt’ explored in our first monograph, Thunderbolts of the Gods.
The Power of Electricity
The free electrons in plasma are about 2,000 times lighter than the protons and are the primary carriers of electric current (they are responsible for the current flow inside a fluorescent light tube, for example).
Wherever electric currents are present in space, they will always pose a challenge to gravity, due to the overwhelming superiority of the electric force. This superiority is seen in the subatomic relationships of the two forces. What is the gravitational attraction between a proton and an electron, in contrast to the electric force between them? As noted in the previous chapter, the electric force is about 1039 times stronger than the gravitational force.45 That means one thousand tril- lion trillion trillion times as strong. And this is why, in the presence of electrical forces within plasma—even when the numbers of ionized
45 “... The ratio of the electromagnetic force to the gravitational force is 39 orders of magnitude.” A. L. Peratt, Physics of the Plasma Universe, p. 48.
Chapter 2 — Plasma & Electricity in Space
atoms may seem insignificant—gravity can be so easily overcome.
In a simple calculation of relative gravitational and electrical forces, the popular physicist Richard Feynman provided a good illustration of our point here. He started with the obvious fact that when two people stand at arm’s length the gravitational attraction between them is so small as to be imperceptible. He then calculated the electrical force that would occur between the two people if their bodies contained only one percent more electrons than protons. His answer: the repelling force between the two human bodies would be sufficient to lift a weight equal to that of the entire Earth!
Another example of comparative strength of gravity and electric- ity is the classic experiment conducted between 1909 and 1917 by Robert Millikan of the University of Chicago, involving a single charged drop of oil suspended in a vertical electric field between par- allel charged plates. Millikan was able to show that a single electron on the oil drop under the influence of a vertical electric field can over- come the gravitational tug on that oil drop created by
the enormous mass of the Earth.
It must be understood, however, that most plas- mas are only partially ionized, the bulk of the atoms remaining electrically neutral. What may seem to be an infinitesimal percentage of charged particles, however, can produce extremely powerful electrical effects. In the dusty filaments from which stars are frequently born, for example, only one atom in
10,000 has lost one or more electrons. But that does not put electric- ity at a disadvantage against gravity, because the electromagnetic forces are incomparably stronger, enabling electromagnetism to dominate the movement and development of structure within these galactic filaments. Even infinitesimal charge separation on a stellar or galactic scale would immediately threaten all of the theoretical edifices built upon gravitational models.
Nevertheless, astrophysicists responded with a further argument.
They made a simple mathematical calculation, familiar to grad students, of how much energy it would take to separate all the electrons from the ions in a teaspoon of salt.
The stupendous sum of energy was greater than anything they could imagine to be available in a gravity-driven universe. Their conclusion was unavoidable: You can’t get charge separation in space. But the question arbitrarily assumes the visible universe is a closed system that ‘began’ with neutral matter. Such is the power of theoretical assumptions in the absence of experiment and observation. Direct observation will pose the question properly: How could a weak force—gravity—generate a universe observed to be swarming with separated ions and electrons? Answer: Gravity cannot be the elementary force behind the structure and movement of the universe.
Nobel Prize winner in Physics, 1923.
Schematic diagram of Robert Millikan’s celebrated oil-drop experiment to measure the charge on an electron. The experiment balanced the phenomenally weak force of gravity between a charged oil-drop and the entire Earth, by the electric tug of a single electron.
Image Credit: Theresa Knott
There is not enough gravitating mass in the visible universe to produce the observed effects of charge separation.
Following the long-accepted view, however, space scientists start with neutral matter. Then they seek to explain how neutral matter becomes ionized and magnetic fields arise. Their models grow increasingly bizarre as space age discoveries reveal complex magnetic fields in unexpected places.
For plasma cosmologists, observations show that charge separation in plasma is the most important state, not neutral plasma. Plasma forms filamentary circuits, current sheets and cellular sheaths. It is the universal reservoir of electromagnetic energy and the medium for its discharge. This universal electric potential is not dependent upon, or generated by, gravitationally driven ‘dynamos.’ That the universe we see and measure is a consumer of electric power, however, is undeniable. So plasma cosmologists study the ways that electric currents produce structure, rotation, and energetic outbursts in the heavens. Fundamentally, they offer a single explanation for what requires many different—and patently insufficient—explanations under accepted theory. In lightning and auroras, in comets, stars, and galaxies, plasma cosmologists can see the signatures of electrical discharge.
The mighty Sandia Laboratories Z- pinch machine. It focuses 80 times the energy of all the power stations on Earth for a billionth of a second into a target the size of a food can. Seen here is the web of filamentary discharges at the moment of firing. Credit: Photo by Randy Montoya (on behalf of US Government laboratory). Courtesy, Sandia National Laborato- ries.
But plasma behavior is complex. Many factors will affect it. For example:
- current density in the plasma;
- dustiness of the plasma;
- electric sheaths formed between plasma re- gions of different character;
- threshold levels at which an electric dis- charge switches to new modes–from ‘winds’ to glows and arcs (see information panel p. 96);
- complex magnetic interaction between cur- rent filaments, forming double helix pairs;
- plasma instabilities;
- and one fact of critical significance: electricity, unlike gravity, can both attract and repel.
By eliminating electrical considerations from their thinking, astrophysicists avoided the more complex numerical modeling required by plasma behavior. In recent years, plasma experts have begun this modeling using the largest supercomputers available, and they have tested these models in plasma experiments at extreme energy densities (see left). They continually cross-check computer simulations with laboratory-generated plasma discharges, utilizing the highest energy conditions that modern technology can produce.
|
A Fateful Turn in Modern Cosmology
Perhaps Kristian Birkeland’s relationship to the scientific mainstream is best illustrated by comparison to the role of another man, the British geophysicist Sydney Chapman. Chapman’s mathematical models of the Earth-Sun relationship appear to have guided mainstream theory through the mid-twentieth century and into the new millennium. He was the acknowledged expert on the kinetic properties of gases (particle motions and associated heat, expansion, and pressure). The equations Chapman and his close colleagues formulated gave numerical values to the ‘kinetics’ of solar behavior and claimed to explain the relationship of this behavior to magnetic storms and auroras on Earth.
Birkeland and Chapman never met, so there was no overt con- troversy between them. Birkeland died in a hotel in Japan about the same time that Chapman’s first papers were published. Birkeland’s experiments with his Terrella implied that charged particles from the Sun are responsible for auroras on Earth. This would mean that something more is involved than such kinetic effects as ‘pressure’ of the solar wind on a self-contained terrestrial magnetosphere. But after his death, Birkeland’s theories were all but forgotten, at least in the short term, while Chapman’s star continued to rise.
In contrast to Birkeland’s experimental work, Chapman’s approach emphasized mathematical clarity. He saw Birkeland’s expensive and dangerous expeditions to the far north as an anachronistic waste of time. It is also said that Chapman, though initially accepting Birkeland’s theory of the auroras, was embarrassed when astrophysicists pointed out that an electric current flowing from the Sun would quickly be stopped by the build-up of electrostatic charge on the Sun. Later he declared that Birkeland was simply wrong. When Hannes Alfvén replicated Birkeland’s Terrella experiment for him, he chose not to examine it.
In the late 60’s and early 70’s, satellites orbiting above the auroras were able to map a surprising movement of ions, proving that electric currents were present. At first these currents were attributed to effects within Earth’s magnetotail, caused by ‘buffeting’ from the solar wind. Chapman still held sway. But later spacecraft found quasi-steady electric fields above the auroras, following the magnetic field lines and confirming Birkeland’s claim of a larger electric circuit between the Earth and the Sun.
Recently, a satellite above Earth’s pole detected electrons streaming from the Earth toward the Sun (right). This unexpected electrical exchange complements the equally surprising discovery of electrons racing from Jupiter toward the Sun, as anticipated by a later electrical pioneer, Ralph Juergens. Such discoveries now verify that electrical currents are active within the solar system.
Sydney Chapman (1888-1970)
Earth and its glowing aurora, with a ‘tail’ of electrons heading from Earth to the Sun. The electron stream confirmed Birkeland’s theory of electrical interactions between the Sun and the Earth. Birkeland suspected that negative particles or ‘cathode rays’ streaming from the Sun caused the auroras, but we now know that the Sun is the more positively charged body in the exchange.
Credit: NASA
INFORMATION PANEL
When it comes to gravitational models, however, testing is usually impossible, and the gravitational cosmologists enjoy a huge window of ambiguity. How does one replicate the imagined dynamics of ‘neutron stars’ or ‘black holes’ or the ‘Big Bang’? Not surprisingly, the ‘explanations’ consistently fail to predict new discoveries in space.
One of the basic assumptions of astro- physics today is that electrical forces play no part in cosmology because “you can’t get charge separation in space.” But X-ray images of space objects tell a different story.
When the Chandra orbiting X-ray camera in December of 1999 looked at a tiny point-source of X-rays embedded in the nucleus of an active spiral galaxy named NGC 4458 (shown in the Hubble image above), it found the region dominated by highly ionized plasma, Many of the elements had been largely or entirely stripped of electrons. All moving plasma produces charge separation and therefore electric currents.
Credit: Optical: NASA/HST; X-ray: NASA/ SRON/CXC/SAO
Power lines that convey electricity across thousands of kilometers from a generator provide a useful analogy to Birkeland Currents in space.
Electric Circuits in Space
From an electrical point of view, circuits extend throughout the visible universe. Radio astronomers detect the intergalactic magnetic fields generated by electric currents on which galaxies are threaded like beads on a string. But they do not realize they are seeing the evidence that electrons are still in the process of finding their partners in galactic-scale glow discharges. No thought was given to the possibility that galaxies and the stars within them experience and are born from a natural charge imbalance.
Alfvén’s life’s work provides the clearest illustration of the theoretical breach that developed between plasma cosmology and mainstream Big Bang cosmology. He began his career as an advocate of an electrically neutral universe. But his change of mind, based on his observations of plasma behavior, would prove a thorn in the side of mainstream opinion. In 1970, Alfvén admonished astrophysicists that they were being deceived by the elegance of their mathematics and that in reality their models are proven wrong by laboratory demonstration. Real plasma, Alfvén said, cannot be treated simply as a magnetizable gas. It is not necessarily electrically neutral, and its response to an electrical current finds no analogy in the other three states of matter.
Cited as the father of plasma cosmology, Alfvén generated volumes of work on the behavior of plasma. And as confirmed in his acceptance of the Nobel prize in 1971 (see p. 10), he recognized that plasma research had moved beyond the earlier theoretical assumptions of mathematicians. He stressed incessantly that the cost of ignoring observations that do not fit theory is extremely high.
Birkeland Currents
Since the mass of an electron is thousands of times less than that of a positive ion, electrons are far more mobile. For this reason, electrons are the major current-carrying particles in plasma. However, they experience a force that deflects them if they attempt to move contrary to the direction of the magnetic force. In this state the electrons get entangled and cannot effectively pass current through the plasma. But if the electrons travel parallel with the direction of the magnetic force, they experience no deflection and no entanglement. Alfvén realized these ‘force free’ or ‘field-aligned’ currents in magnetized plasma could be astronomically significant.
Chapter 2 — Plasma & Electricity in Space
Alfvén recognized that such currents could form invisible cosmic transmission lines, pumping electrical energy from one part of the cosmos to another. The principle is analogous to electric power distribution on Earth. In fact, the structure adopted by plasma current filaments is comparable to the multi-stranded wire of transmission lines. When electric current flows in alignment with the magnetic field, it is ‘pinched’ into long filaments by the magnetic forces associated with the current itself. This is called the ‘z-pinch effect’ because the magnetic field direction is conventionally assigned to the z-axis in a three-dimensional representation. The filaments then twist themselves into rope-like structures called ‘Birkeland currents.’
Thanks to the Hubble telescope, what Birkeland and Alfvén described as electromagnetic forces organizing galaxies can now be seen in the spiral forms, axial jets, and filamentary structures of galaxies, nebulae, and comet tails. These twisted current-ropes are the tracers of electricity, forming repeated patterns at all scales of observation. Also, the high-energy stellar and galactic patterns recently revealed by the Chandra X-ray telescope add further support to Birkeland’s and Alfvén’s claims that electric forces drive the cosmos. The Chandra X-ray images are especially revealing to plasma cosmologists, for X-rays are found wherever powerful electrical discharges occur.
As remarkable as it may seem, however, most astrophysicists today have never heard of Birkeland currents. Only a restricted form of plasma physics—magnetohydrodynamics—is being taught to them. Astronomy textbooks will typically deal with plasma in a few sentences, perhaps mentioning the Earth’s ionosphere, comet tails, or the solar wind—all explained in traditional kinetic or mechanical terms and ignoring the unique electrical properties of plasma and plasma discharges. International conferences on plasma cosmology generally go unnoticed by mainstream astronomical journals, and only a small number of high voltage engineers and plasma physicists have sufficient experience to address with authority the complex, almost lifelike behavior of discharge phenomena in plasma.
Both Newton and Einstein lived in the .001 percent of the universe that is not plasma—at the bottom of the atmosphere of a rocky planet. So it is not surprising that they did not take plasma behavior into account. Since it is now accepted that we live in a plasma universe, however, ignoring the electrical properties of plasma is not a minor oversight!
Today the symptoms are too obvious to be denied. From the scientific mainstream, expressions of surprise come far more frequently than any confirmations of theory. The list would include: never-imagined galactic structures in deep space; coherent filaments and related magnetic formations spanning hundreds of light years; stars with polar jets punctuated by star-sized knots or matter
Electric currents in plasma naturally form filaments due to the squeezing or ‘pinch effect’ of the induced magnetic field. Electromagnetic interactions cause the filaments to draw inward and rotate about each other to form a helical ‘Birkeland Current’ filament pair, or plasma vortex.
|
When Gravity Doesn’t Work
Something strange is happening to the space probes Pioneer 10 and 11, causing quite a stir among physicists. Precise tracking of these craft, launched in 1972 and 1973, has shown that they are mysteriously slowing faster than the law of gravity predicts. By our everyday standards the discrepancy—just six m.p.h. per century—is minimal. But this divergence seems constant with distance from the Sun and it puzzles the experts. Are ad hoc modifications of the law of gravity necessary at large distances? Is the deceleration somehow linked to the imagined ‘expansion of the universe?’
Some have also wondered, if this mysterious behavior were understood, might it help to explain the even more mysterious rotation of spiral galaxies? Spiral galaxies do not obey Newton’s law. They rotate more like a solid plate, with the same galactic ‘year’ for most stars in the galaxy. (Just imagine every planet in the solar system revolving around the Sun in one year!)
Plasma physicists are not dismayed by the flat rotation curve of galaxies. Decades ago they showed that the forms taken by spiral galaxies can be reproduced in their laboratories when powerful electric currents intersect in a plasma. Given the recent discovery of ordered magnetic fields—the signature of electric circuits—within these galaxies, the implications of the earlier research are now obvious. But rather than accept the possibility of long-range electromagnetic forces in space, theorists envisioned
a huge halo of invisible matter outside the visible galaxy, forcing the stars within to rotate as a rigid plate. From this reasoning they concluded that more than 90 percent of the matter in the universe must be invisible ‘dark
matter.’ “So why should I bother looking through a telescope,” one astronomer mused, “if 90 percent of whatever is out there can’t be seen?”
But there is good reason to continue looking through telescopes, as challenges to gravity’s supremacy grow every year. Instead of a universe made up largely of undetectable ‘dark matter’ we should better understand the detectable universe, which is made up of 99.99 percent plasma.
ABOVE: M51, The Whirlpool Galaxy Credit: NASA (STScI/AURA)
BELOW: A Herbig Haro object — HH111.
It sports an obvious helical structure in its 12 light- year long jet, characteristic of a Birkeland current. The jet requires electrical energy input to light up the plasma and maintain the jet at great distances from the star.
Credit: NASA & B. Reipurth CASA, University of Colo- rado).
INFORMATION PANEL
|
What is the ‘Electric Universe?’
The ‘electric universe’ is a hypothesis, a new way of interpreting scientific data in the light of new knowledge about plasma and electricity. In this inter- pretation, gravity plays a secondary role behind the far more powerful electric force. And electrified plasma in the laboratory provides a model for comprehending newly-discovered phenomena in space. As laboratory plasma ‘pinches’ into filaments, sheets, and cells, or isolates charged bodies from
their electrical environment, it provides vital clues. In plasma, electric currents will sort material into shells of like elements, or generate dis- charge patterns— ranging from radial streamers and spi- raling filaments to exotic symmetrical configura- tions—all appearing to mimic what we now see in faraway corners of the cosmos.
Our centerpiece on this page is a Hubble image of the planetary nebula NGC6751.
The experimental counterpart is
provided by a plasma focus device (below), which concentrates electrical energy in an explosive dis- charge (below right), mimicking the structure of NGC6751 down to certain telling details. In contrast, gravitational models neither anticipated such configu- rations nor offer plausible explanations.
In the electric universe the gravitational systems of planets and moons, stars, and galaxies have their origin in this proven ability of electricity to generate structure and rotation in plasma. Gravity can take over only as the electromagnetic forces approach equilib- rium.
The extraordinary configurations now seen in space are the result of electric currents, where gravity
cannot compete with the electric force. Such struc- tures as NGC6751 are telling us that something is funda- mentally wrong in the ap- proach taken by astrophysi- cists. It is no longer tenable to build a cosmology on the idea that neutral matter is the ‘starting point.’ All varia- tions on that theme will re- quire gravitationally-driven generators to ‘separate charge.’ But it is not logical to ask the weak force of gravity to produce the strong
electric force from which gravity-defying motions arise in galaxies. Direct ob- servation implies that electrified—not neutral—matter, is the fundamental state of the universe.
To be sure, our human perspective is limited and the origin of the universal electric power grid will likely remain as elusive as the origin of matter in the universe.
View down the barrel of the Plasma Focus
INFORMATION PANEL
concentrations; numerous electrical and magnetic attributes of our Sun; X-ray emissions from comets and other evidence of electrical effects governing the behavior of comets, and vast networks of strange scars on planets, moons and asteroids. But in response to these and other surprises the gravitational theorists have added increasingly complex explanations and exceptions. The result is a tangle of exceptions far more numerous than the original ‘rules.’ For anyone wishing to disentangle fact from assumption, this may seem a nearly hopeless situation.
And yet, at root, the situation is radically simplified by simply allowing for the obvious. Electric fields are extremely active in the cosmos.
A celestial Birkeland current. The Double Helix nebula near the galactic center.
Credit: NASA/JPL-Caltech/UCLA
Electricity and Magnetic Fields
The discoveries of the space age have come so rapidly that it is easy to forget how recently they altered our vision of the heavens. In 1950, prior to the space age but centuries after Newton, the term ‘magnetosphere’ had not yet entered the lexicon of astronomy.
Astronomers never anticipated the intricacy of magnetic fields surrounding the Sun, or the great webs of magnetic fields now recognized in deep space. But today one fact is beyond dispute: the more we have learned about objects in space, the more magnetic fields have risen to prominence.
Magnetic fields arise from electric currents. How, then, have astrophysicists preserved their gravitational models in the face of electricity’s obvious presence throughout the universe? They generally responded by imagining mysterious ‘dynamos,’ situated conveniently out of sight, inside celestial bodies. Or they simply confess that “the origin of the magnetism is not yet fully understood.” However generated, the magnetic fields are magically trapped in ‘superconducting’ plasma that occurs nowhere in space.
Though our summary here may seem harsh, it is in fact an accurate statement of the situation. A failure of reasoning has occurred within astronomy, and the consequences grow more severe each year. All astrophysicists now acknowledge the presence of vast magnetic fields in space, but they are trapped in a gravitational cosmos, one that can be preserved only by resorting to a wholly disproved assumption—that plasma can be treated as a superconductor, immediately short- circuiting any charge imbalance. On this reasoning magnetic fields can be ‘frozen’ or ‘trapped’ in plasma, persisting indefinitely.46
Abstract deductions led to a fundamental mistake, exposed both by laboratory experiments and discoveries in space. Plasma is indeed a far better conductor of electricity than copper, but it is not a
46 Or until the energy stored in the field is released in a mysterious process called ‘magnetic reconnection.’ See p. 64 for a discussion of this creative fiction.
Chapter 2 — Plasma & Electricity in Space
superconductor. It has a characteristic impedance of about 30 ohms, and wherever there is impedance the energy held in the magnetic field will be dissipated in the form of electromagnetic radiation, such as light, heat and radio waves. For this reason, radio telescopes have provided a marvelous boon to astronomical discovery, though mainstream astronomers themselves initially opposed them.47 But we now know that powerful radio transmissions from cosmic sources are extremely common; and they are a predictable effect of electric currents, as energy is dissipated in stellar and galactic circuits. A source of electric current is thus required to sustain the magnetic fields detected around stars and galaxies.
Here we must highlight the fact that plasma in outer space is extremely tenuous, with perhaps one particle per cubic centimeter, compared to 1013 particles per cubic centimeter in Earth’s atmosphere. The less dense the plasma, the less current it can carry per unit
volume, despite the fact that it is an excellent conductor. Since plasma in space is more diffuse than in any vacuum that can be produced on Earth, the limits of its current-carrying ability can hardly be ignored. But as noted in the following pages, while current density across the vacuum of space is extremely low, the mind boggling volume of space, together with very weak electric fields, easily and efficiently delivers the currents that power stars and galaxies.
Do Planets Carry Electric Charge?
Abundant evidence confirms that planets do, indeed, carry charge, but the issue is often susceptible to misunderstanding. How do we measure the voltage of a planet? Voltage difference is relative, and measuring it requires a reference point. A bird sitting on a thousand
BELOW LEFT: a supernova remnant, Cygnus Loop. Its aurora-like curtains and filaments have far more in common with electric currents in plasma than with mechanical models based on ‘acoustic shocks.’ Electrical activity will also sort chemical elements into discrete regions, as seen in the color coding of Cygnus Loop according to element.
Other characteristics of the Cygnus Loop include polarization of light, filamentation, acceleration of relativistic electrons, and X-ray hotspots—all expected of electrified plasma.
Credit: NASA/, HST, WFPC2, Jeff Hester
BELOW RIGHT: Close-up of a Cygnus Loop Birkeland current. It is an un- derstatement to say that neutral gases do not take such form in a vacuum.
Credit: William P. Blair and Ravi Sankrit (Johns Hopkins University), NASA
47 “Although Karl Jansky discovered the existence of radio emissions from outer space as early as 1932, a decade passed before the scientific world began to take an interest in it.” Editor, Proceedings of the IRE, Jan 1958.
Planetary nebula NGC 6826 shows many electric discharge phenomena associated with a star under abnormal electrical stress. The bubbles or plasma cells nearest the star glow brightly because of the strong electric field across each cell wall. Electric currents flowing to the star show the usual filamentary structure of Birkeland currents. The red tufts, known as FLIER’s (fast low-ionization emission regions), result from polar jets from exploding double layers in the polar circuit
driving the central star. It is important to realize that the energy radiating from the nebula is not provided by the star but is electrical energy intercepted on its way through the stellar circuit, to and from the star.
Credit: B. Balick, J. Alexander (University of Washington), et al., NASA
volt wire is unaware of it unless a wing touches a nearby wire at zero volts. On our planet the ‘zero-volt’ reference for measuring the voltage of an object is the earth itself, sometimes called the ‘ground.’ But like the bird on the high-voltage wire, we would be unaware of, say, a charge generating a billion-volt difference between the Earth and the Moon. We don’t have a voltmeter with leads long enough to stretch between the two bodies to determine if this differential exists. And we cannot simply measure an electrostatic field between the Earth and the Moon because plasma conspires to form an insulating cocoon or sheath about charged bodies.
So could we answer the question by sending spacecraft to the Moon? In this case the spacecraft, through the course of its journey, would exchange charge with the surrounding plasma. So when it arrived it could no longer be used as a reference to measure the Moon’s voltage with respect to the Earth.
The ability of a spacecraft to adjust to the charge of its surroundings, however, is limited. The more rapidly the spacecraft traverses regions of different potential, the more likely that insulating materials in the spacecraft will charge to a level leading to arcing within the craft, or from the craft to the plasma in its immediate vicinity. Based on difficulties actually experienced in transit, including damage to instruments and to spacecraft, NASA has done studies on how interactions with the surrounding plasma affects space probes and space vehicles—though the studies have not explicitly addressed the issue of planetary charge.
One study showed that discharges within a spacecraft “can produce a result to satellite operations that is not unlike a…cosmic- ray- or proton-induced single-event upset. Typical earth orbiting spacecraft stop enough charge to develop threatening electric fields in the insulated components within minutes to weeks after launch.”48
However, instruments are rarely built into spacecraft to provide meaningful data on internal electrical discharges, so many issues remain conjectural. Also, even if plasma were charge neutral, the faster electrons would hit a spacecraft more often than the slower ions, charging it up negatively with respect to the surrounding plasma. The few measurements that have been done show that the voltage difference can be as high as ten thousand volts. “Spacecraft operators are unaware that pulsing [electrical discharges] on one spacecraft may only be noise, but the same plasma bursts on the next spacecraft may short a power supply.”49
In a multi-billion dollar, tax-supported space program, ignoring
48 A R Frederickson, ”Upsets Related to Spacecraft Charging,” IEEE Transactions on Nuclear Science, Vol 43, No. 2, April 1996, p. 426.
49 Ibid., p.433.
|
Langmuir Sheath and ‘Magnetosphere’
Understanding what happens to an object embedded in plasma is essential to an appreciation of the electric forces at work in the universe. Late in the nineteenth century, Sir William Crookes (1832-1919) introduced metal probes into the plasma of an electric glow discharge to measure the electric fields there. This was the method Langmuir used, but he discovered that the probe itself would become negatively charged and prevent measurement of the plasma potential. The much lighter and faster electrons charge the probe negatively until this charge is strong enough to limit a further flow of electrons.
The accompanying graphic illustrates the different conditions affecting the Langmuir sheath. The probe (b) is disconnected from an electric circuit. Here the accumulating negative charge attracts ions and repels electrons to form a positive space charge sheath, called a ‘Langmuir sheath.’ Its radial electric field ‘protects’ the surrounding plasma from this foreign charged body. An example of an isolated probe is a spacecraft like Pioneer 10, shown on its way to the stars. Such spacecraft are known to charge up negatively in space relative to their surroundings.
Langmuir invented a plasma probe in the 1920’s, subsequently named after him, that allows indirect measurement of the plasma potential, the electron-ion concentration, and electron temperature. The Langmuir probe is connected to a variable voltage source, and current flowing through the probe is plotted against voltage as shown in the diagram. The true plasma potential can be deduced from this plot. The Langmuir sheath disappears when the probe is at plasma potential (c) and the currents due to random motions of ions and electrons in the plasma are equal. The Moon is an example of such a body, in direct contact with the solar plasma.
If the probe is much more negative than the plasma, as in (a), the positive Langmuir sheath will
expand. Although the magnetosphere of the Earth has many complicating features, its size is primarily due to a negative voltage on the Earth compared to the Sun’s plasma. Venus has a small magnetosphere, and Jupiter’s is huge, offering crude measures of voltage differences between the planets and the solar plasma.
From this simple picture, a question arises. The probes (a), (c) and (d) are connected to an external circuit. Where, then, is the ‘external circuit’ for the Sun or the planets within the Sun’s system? The circuit is galactic. The Sun, therefore, is comparable to positively charged probe (d). In addition, there are many complex plasma discharge phenomena to be explored concerning the Sun, including a new perspective on how it formed and how it works today. We begin this investigation in Chapter 3.
INFORMATION PANEL
The schematic above illustrates the electric field within the insulating sheath that develops around a charged body in a plasma. White signifies the charge on the body, and black the charge of plasma through which it is moving.
experimentally based plasma behavior can be costly. Among the known failures are these: the Pioneer probes to Jupiter were effectively crippled by unexpected ‘radiation.’ The Galileo probe lost a portion of its images and data acquisition
due to ‘safing-mode’ shutdowns of onboard equipment at critical times, usually on closest approach to Io. Mars probes may have been especially susceptible. Of the more than two-dozen spacecraft that left Earth orbit en route to Mars, two thirds came to the end of their missions prematurely, for unknown reasons. How many of these were due to unanticipated electrical effects? Closer to home a disastrous Tethered Satellite System experiment in space in February 1996 must also be mentioned. After 19 of the 24 kilometers of the conducting tether was released from the Space Shuttle Columbia, the wire was mysteriously burned through by an electrical discharge. Before that the tether was producing at 400 volts the current expected at 7000 volts.
The experimenter in charge noted, “It’s quite a disparity.” It is probable that such tethers and ‘space elevators’ are technologies that will not succeed in their present form because the science behind them does not take account of the Earth’s electrification.
Sadly, NASA has never adequately investigated the possibility, claimed by some, that the shuttle Columbia was lost in 2002 because of a high-energy ‘bolt from the blue’ that was photographed but explained by NASA as a photographer’s error (the photographer is an experienced amateur astronomer).50 NASA engineers caught the blame for an accident that may, in fact, have been a failure of NASA scientists to understand the electrical nature of the Earth. They discounted the possibility of a plasma discharge because the Shuttle wasn’t flying above a thunderstorm at the time! However, a solar storm reached the Earth at that time. And auroral type discharges51 are known to follow coastlines preferentially.
If electricity is ‘out there,’ why can’t we measure an electric field linking the Earth and other bodies in space? The strength of an electric field is usually measured in volts per meter, but the behavior of plasma
50 S. Russell. San Francisco Chronicle, Feb 5, 2003. “S.F. man’s astounding photo: Mysterious purple streak is shown hitting Columbia 7 minutes before it disintegrated.” See:
www.sfgate.com/cgi-bin/article.cgi?f=/c/a/2003/02/05/MN192153.DTL&type=science.
51 P T. Newell et al., “Relation to solar activity of intense aurorae in sunlight and dark- ness,” Nature Vol. 393 (1998), pp. 342-4. “These findings imply a causal relationship between aurorae and ionospheric conductivity and therefore indicate that the occur- rence of intense aurorae is a discharge phenomenon, similar to lightning.”
|
Discovering the ‘Magnetosphere’
In a basement lab of the physics department at the University of Iowa, James Van Allen designed Explorer 1, a scientific satellite for the International Geophysical Year (IGY) of 1957-1958. The satellite carried only a small Geiger counter. The instrument was designed to measure cosmic rays, highly energetic ions (positively charged particles) of
unknown origin arriving from distant space.
Strangely, the experiment detected many particles at low altitudes, but at the top of the orbit it counted no particles at all, The explanation was discovered two months later by Explorer 3. This new satellite included a tape to record data continuously.
It revealed the ‘absence’ of cosmic ray counts from Explorer 1 actually signified extremely high radiation. “So many energetic particles hit the counter at the higher altitudes that its mode of operation was overwhelmed and it fell silent,” states a NASA account. “Not only was a radiation belt present at all times, it was remarkably intense.”
Scientists had discovered the innermost of the two Van Allen Radiation Belts— hailed as the first important discovery of the space age. It could also be called the first surprise of the space age. Had physicists paid more attention to Birkeland’s experiments it need not have been a surprise.
Birkeland’s Terrella experiments demonstrated most of the phenomena found by spacecraft near the Earth. But for scientists the stumbling block was that the Terrella required electrical power input to the Earth, and standard astrophysics has no mechanism to support such a model.
We now know that the Earth is surrounded by a complex structure of magnetic fields and high-speed charged particles that include streams of electric cur- rent around the Earth. This structure has been named the ‘magnetosphere’ under the assumption that it forms the boundary between the Earth’s and the Sun’s magnetic fields.
However, comets form similar protective sheaths, visible as their comas, without having magnetic fields. In a plasma discharge most objects naturally form a Langmuir plasma sheath that prevents direct contact with the enveloping plasma. Magnetospheres are merely a more complex form of Langmuir sheath, with the magnetic field of the body trapped within the sheath. If standard theory failed to anticipate these discoveries, surely the primary reason lies in the failure to recognize that planets are embedded in a solar electric discharge and consequently enveloped by Langmuir sheaths. For these reasons, the original contributions of Kristian Birkeland can no longer be ignored.
ABOVE LEFT: The first successful U.S. satellite, Ex- plorer 1.
Credit: JPL, NASA
ABOVE: Standard illustration of the Earth’s ‘magneto- sphere’ in its relationship to the ‘solar wind.’ Failure to recognize the Langmuir sheath has led to many misconceptions.
Credit: NASA
INFORMATION PANEL
The traditional view of the solar system sees planets, moons, and asteroids moving in isolation through a vacuum. A more sophisticated view considers the heliospheric current sheet illustrated above, a component of the interplanetary plasma that pervades the solar system.
Credit: Image developed by Prof. John Wilcox from an original painting by NASA artist Werner Heil
does not permit us to simply divide voltage by distance.52 It is essential, therefore, that the plasma’s response to embedded charged objects be clearly understood.
The use of the term ‘plasma’ began in 1927 with Irving Langmuir. In his work for the General Electric Company, studying electronic devices based on ionized gases, Langmuir noticed that the way the electrified ‘fluid’ carried high velocity electrons and ions was similar to the way blood plasma carried red and white corpuscles. He showed that when a charged object is introduced into a plasma, the plasma self-organizes to isolate the foreign object electrically by producing a cellular formation around it, now called a ‘Langmuir sheath.’
This characteristic of plasma means that if the Sun, planets, and other bodies in space possess potentials different from that of the surrounding plasma, the first thing to look for would be evidence of Langmuir sheaths around them. A second thing to look for would be a weak electric field between the object and its sheath boundary. A third would be a strong field within the boundary or ‘membrane’ (known as a ‘double-layer’) of the sheath.
If our Sun is a charged object, for example, then its Langmuir sheath will be the ‘heliosphere,’ the vast comet-like cell enclosing the Sun and all of the planets, that isolates the system from the larger galactic environment. Voyager 1 reached this ‘edge of the solar system’ in December 2004 in a region 94 times more distant from the Sun than the Earth. It is a region where it was expected that the ‘supersonic solar wind’ would suddenly slow down when it met the interstellar medium at what is called the ‘termination shock.’ This
fluid-mechanical analog is vastly different from the electrical model, in which the Sun is a ‘unipolar’ corona discharge. That is, the Sun forms the physical anode (positively charged object) and the plasma itself performs the function of the cathode (negatively charged object). High voltage transmission lines on Earth undergo a similar ‘corona discharge’ into the surrounding air, which forms a ‘virtual
cathode’ (not an object, but a region of charge). In this model, almost the entire voltage difference between the Sun and its galactic environment–estimated to be tens of billions of volts–will be concentrated at the virtual cathode boundary with interstellar space. So it is only to be expected that the data from Voyager 1 is anomalous for
52 Also, the mere act of measuring the field by the force it exerts on a charged particle will disturb the field, so even direct measurement can be difficult. Indirect means of measurement are therefore often necessary, and lay themselves open to the assumptions made by astrophysicists about the nature of the plasma. That is one of the reasons why astrophysicists can deny that the Sun has a radial electric field.
the magnetohydrodynamic ‘shock’ model53 while it seems consistent with the electrical model. In the electrical model, Voyager 1 has entered the ‘Faraday dark space’ region of the solar discharge where the solar electric field is reversed. That would account for the pile-up of the protons in the solar wind and the steady increase in anomalous cosmic rays from a more distant region.
Within the heliosphere, the higher density plasma near the Sun must give rise to one or more plasma sheaths above the surface, one of them being in the Sun’s chromosphere. In this electrical
profile, the Sun’s chromosphere must be a region of high field strength
—incomparably higher than the field strength in the vicinity of the Earth, for example. As noted in Chapter 3, this explains the thermodynamically impossible multi-million degree corona above a cool photosphere.
This electrical model also offers a simple answer to the perennial puzzle of the origin of most cosmic rays. Every star in the galaxy will have its own huge positive ion accelerator.
If planets do indeed carry charge, then Langmuir sheaths must be present around the Earth and the other planets as well—the secondary plasma cells within a larger solar plasma cell. In fact, the planets do possess Langmuir sheaths, in the form of teardrop shaped structures. On a planetary scale, discovery of these insulating bubbles with their enclosed magnetic fields prompted astrophysicists to call them ‘magnetospheres.’ They assumed that these cells were simply plasma trapped in a planetary magnetic field and shaped by the supersonic solar ‘wind.’ From an electrical viewpoint, a more appropriate term would be ‘plasmasphere,’ or ‘plasma cell’: the planet’s electric and magnetic field are trapped inside a Langmuir sheath.
It is the lifelike and cellular behavior of plasma that creates the inherent difficulty in measuring the electric fields of the planets and of the Sun. Moreover, the use of indirect means will be open to interpretations based on the assumptions about the nature of plasma.
Earth’s magnetosphere. The complex environment in space around the Earth was given the name ‘magnetosphere’ in 1959 by Thomas Gold of Cornell University. In the electrical model we may see it as a Langmuir sheath, distorted from spherical symmetry by the Earth’s magnetic field and the solar wind traveling past at hundreds of km/sec. Credit: ESA
53 L. A. Fisk, “Journey into the Unknown Beyond,” Science, Vol. 309, pp. 2016-7. It was assumed that the termination shock would provide the energy for the acceleration of anomalous cosmic rays (ACR’s) and would provide an accessible example of how supernovae are supposed to produce galactic cosmic rays. However, there is no evidence of this mechanism. Once again the mantra of space exploration is fulfilled: “When we go somewhere that is new, we find the unexpected..”
Terrella experiment, illustrating the interaction of the solar wind with the Earth’s magnetic field. The ‘solar wind’ source is a plasma thruster (LEFT). The trapped radiation belts are to scale, with a picture of the Earth superimposed to scale on a bar magnet.
The irony is that the plasma thruster (representing the Sun) requires electric power! (Picture courtesy G. R. Seikel, NASA Lewis Research Center, Cleveland, Ohio.)
When astrophysicists assume that the magnetic field is ‘trapped in superconducting plasma’ they believe they can ignore the implied electric field. But as Alfvén (and today, numerous others) point out, that is a costly mistake.
Once such ‘cells’ are recognized as Langmuir sheaths the picture changes completely. Then these cells become the direct evidence for electricity on an interplanetary and interstellar scale—electricity with the power to shape galaxies, to energize stars, and to overwhelm the forces of gravity when two plasma cells interact. Indeed, these Langmuir sheaths do not just imply charged bodies in space; by their size and electrical activity they also give us clues for estimating the relative strengths of the electrical charges.
If the Earth is electrically charged, there should be a detectable electric field at the surface. And there is. In fair weather, this electric field measures about 100 volts per meter as you move up from the surface. But here, too, it would be a mistake to draw quick conclusions based on simple electrostatic arguments. This measurable potential can tell us very little about the Earth’s charge, since it only represents a miniscule sample of the total potential from the surface of the Earth to the solar plasma beyond our planet’s Langmuir sheath. Most of the voltage drop must occur within the sheath membrane or ‘double layer,’ forming the boundary isolating the Earth’s plasma domain from that of the Sun.
Langmuir Sheaths and Auroras
When the plasma environment of our Earth changes, as occurs dramatically during a solar storm or coronal mass ejection (CME), the configuration of the Earth’s Langmuir sheath changes in response. It can expand, contract, or flutter. For the charged Earth within its plasma sheath, this can mean a multitude of familiar effects, including storms, lightning, auroras, and widespread power blackouts.
If the Earth is charged, we should expect electric currents to flow
between the Earth and space. Moreover, these currents should be directly connected to, and affected by, the electric ‘wind’ or flow of charged particles emitted by the Sun and accelerating past the Earth. Today, after nearly a century of debate against Birkeland and Alfvén, mainstream science accepts that outbursts of charged particles from the Sun are the cause of the aurora. Auroral activity is now forecast on the Internet like ordinary weather. But how does this effect occur?
At issue is the relationship of the Earth’s Langmuir sheath to the surrounding plasma domain of the Sun. Physicists, using the language of traditional
dynamics, envisioned Earth’s ‘magnetosphere’ as a windsock waving in the ‘solar wind.’ Since kinetic effects are certainly present, this language is not entirely inappropriate. But neither is it sufficient. To the side facing the Sun they gave another mechanical expression, ‘bow shock,’ because it reminded them of the deflection of air around a supersonic aircraft. They argued that this bow shock compressed the Earth’s magnetic field lines and deflected the ions of the solar wind, preventing these charged particles from entering the magnetosphere.
In a charge-neutral planetary system, no electrical transaction will
occur between the separate parts. The magnetospheric bubble can only be pushed, stretched, or compressed by the ‘pressure’ acting on it from outside, and nothing internal to the magnetic bubble is exchanged with the external ‘wind,’ except mechanical energy resulting from the kinetics of particle motions. From this vantage point, the theorists could only explain the electrical responses of Earth (increased lightning activity and auroras, for example) as the conversion of mechanical energy into magnetic energy in the Earth’s field, subsequently released in the form of electric currents within the ionosphere.
NASA image of aurora australis taken from the Space Shuttle in May 1991. It shows typical filamentary and sheet glow discharge effects.
Credit: NASA/JSC.
LEFT: Examples from Birkeland’s Terrella experiments with artificial auroras, produced by means of an electric discharge to a magnetized sphere suspended in a large evacuated glass container.
Compare the satellite photograph of Jupiter’s powerful aurora (below).
Credit: NASA/J. Clarke (University of Michigan)
So when researchers first discovered high-energy solar wind ions inside the Earth’s magnetosphere, it baffled them. How did charged particles streaming past the Earth’s poles move at right angles across magnetic field lines and penetrate into the Earth’s protective magnetic bubble?
In truth, any attempt to mimic the auroras through physical models will require a source of electrical power. If Earth is, in fact, at a nega- tive potential in relation to the Sun, it is only to be expected that an electrical disturbance of the Sun should produce the very effects we observe—enhanced current flows in the form of solar wind ions cross- ing Earth’s plasma sheath, and jets of electrons leaving the Earth above the magnetic poles. Auroras and electrical storms on Earth are linked to solar activity in the same way that a lightning strike on a power line will invariably affect consumers down the line. The electrical systems of the Earth and the Sun are connected.
Photograph of a lightning sprite above a thunderstorm.
Credit: University of Alaska Fairbanks
Lightning Sprites and Their Companions
Like auroras, many aspects of terrestrial lightning remain mysterious only because the larger electrical circuitry goes unnoticed. If the Earth is continually interacting with an external electric field, then terrestrial lightning surely involves something more than wind-driven charge separation in storm clouds in a circuit restricted to the lower atmosphere. In recent years it has been found that lightning storms are often accompanied by strange faint flashes, playfully called elves, sprites, and gnomes, radiating into space high above the clouds. This is precisely what we should expect if lightning completes a much larger circuit extending into interplanetary space.
Few direct measurements have been made of sprites. The thin mesosphere where they appear is too high for research aircraft
to fly and too low for orbiting spacecraft. Most of what’s known has come from low-light video cameras and electromagnetic sensors housed on mountain-tops in stormy regions. Recently, high-altitude
balloons have provided a closer look at sprites, and what they saw not only shocked the scientists involved, but confounded all standard ex- planations of these mysterious phenomena.54
The leader of one high-altitude balloon team, Edgar Bering, of the University of Houston Physics Department, found that sprites are several times more powerful than expected. This strength is predictable, however, if sprites are the means by which current is transmitted to the region below, energizing the more visible and familiar lightning display seen from Earth.
One of the big surprises was the discovery that sprites are
54 H. Williams, “Rider on the Storm,” New Scientist, 15 December, 2001, p. 40.
produced by a sudden burst of current, instead of a slow build-up of an electric field. It had always been supposed that heat and atmospheric turbulence in a cloud, by means “not yet fully understood,”55 separate electrical charge, then release it through lightning discharges.
The sprites show that the earlier suppositions are incorrect. Bering found that the sprite discharged only milliseconds after the lightning beneath it, and the instruments picked up no signal of a slowly building electric field before the discharge. To a plasma cosmologist, the finding implies that lightning completes an electrical pathway from the surface of the Earth to space, in effect ‘throwing a switch’ to light up the huge sprites above.
Bering’s own conclusion could not have been more emphatic: “None of the existing models will survive when people finally pay attention to what our data actually says.”56
If the Earth is a charged body connected to the Sun’s electric field, then the storm, the lightning and the sprites will be manifestations of a single phenomenon, and the mystery is immediately solved. Lightning is the spark of a celestial current as it connects to the Earth. The situation does not require theoretical guesses as to the mechanics of charge separation, only a mechanism for charge recombination—or electrical discharging—a requirement that is met by the electrically driven, rapid vertical movement of air in a storm that typically precedes the lightning stroke.
With this understanding, the difference between the appearance of lightning below and above the clouds is simply a matter of atmospheric density. The very low air pressure at great heights will affect the size and color of the sprites, an effect well illustrated in laboratory experiments. Within a vacuum tube, a long discharge spark
—the analogue of a lightning stroke—will begin to produce colorful glows and filaments—the analogue of sprites—when most of the air is removed.57
What the sprite demonstrates most persuasively is that the earth is not an electrically neutral body in space. The sprite shows that the circuit stretches high above the clouds toward space. Thunderstorms are not electrical generators, they dissipate energy in an interplanetary circuit, like a self-repairing leaky capacitor, familiar to electronics engineers. The charge across the cloud ‘capacitor’ gives rise to
55 M. A. Uman, Lightning, Dover 1984, p. 2.
56 H. Williams, “Rider on the Storm,” New Scientist, Vol. 172 No. 2321, 15 December 2001.
57 E. R. Williams, “Sprites, Elves, and Glow Discharge Tubes,” Physics Today, Vol. 54, No. 11, November, 2001, pp. 41-5. “The venerable field of gaseous electronics underlies the understanding of a lightning-like phenomenon of spectacular extent, shape, and color. Sprites and elves are a grand natural manifestation of ideas and laboratory experiments conceived many decades ago by Rayleigh, Thomson, Wilson, and Langmuir--all of whom won Nobel prizes--and by a host of 19th century glow dis- charge tube spectroscopists.”
Artist’s depiction of the lightning forms occurring above a thunderstorm. These discharge configurations can be compared to plasma formations in the laboratory— filamentation, corkscrews, ropes, beading, and toruses.
Credit: New Scientist, W. Thornhill
ionization and violent vertical electrical winds within the cloud. Thus, the positive cloud-to-ground lightning from a cloud top simply creates a ‘short-circuit’ to high altitudes in the storm, where the glowing form of the discharge—the sprite— flashes in the upper atmosphere.
What, then, must be occurring above the sprites, to confirm that these electric currents extend into space? In 1998, a Stanford University group discovered that some lightning strikes are followed about a second later by changes over enormous areas in the conductive plasma regions of the ionosphere, which begins about 85 kilometers above sea level. In particular, one lightning strike over Austin, Texas, was followed by a disturbance of the ionosphere above a large region from Kansas to South Dakota, including most of Iowa. The ionosphere is well above the atmospheric layer where sprites appear, and the measured disturbances revealed currents flowing from the even higher Van Allen Radiation Belts.
Therefore, it can no longer be doubted that the electrical events we see in thunderstorms are inseparable from electrical events far above the clouds. In fact, every time we’ve found a way to look higher, we’ve discovered more electrical activity. Have we now discovered the ‘top’ of the circuit? In an electric universe there is no end to electrical connectivity. Electrically isolated bodies do not exist.
By attempting to explain lightning through the behavior of water droplets in turbulent thunderclouds, atmospheric physicists have always considered lightning to be associated with water clouds. But lightning has also been discovered on Venus, which has no water at all in its searing hot smog. (As one NASA scientist exclaimed, “You don’t get lightning in
smog.”) Lightning occurs on Jupiter, and (as noted below) it is extremely active on the Jovian moon Io, which has no atmosphere. By placing lightning in its larger context, the electric universe has no need to add new mechanisms to explain lightning each time it is discovered elsewhere in the cosmos.
A Matter of Viewpoint
How did such radically different theoretical perspectives arise? As plasma physicists look at astronomy and astrophysicists look at plasma, the respective languages, cultivated over many decades, can only accentuate the gap in viewpoints. For plasma physicists the focus is on the movement of electric charge. From lab experiments, they know that a few charged particles in a gas can produce electromagnetic effects that dominate mechanical effects. Equations used by plasma cosmologists come from electrodynamics and circuit theory. Their
|
Earth—the ‘Self-Repairing Capacitor’
One electrical device which serves as a model for cosmic plasma sheaths is the capacitor. A capacitor is a device for accumulating and storing electric charge. It is made of two conductors separated by an insulating medium. The first capacitor was the Ley-
den jar (see page 7), used in static electricity experi- ments. When charge is placed on one conductor it attracts charge of the opposite polarity on the other conductor. As a result, an electric field is set up be- tween the conductors, a reservoir of electrical en- ergy. In both everyday electronics and advanced plasma research the capacitor is important for
its ability to rapidly store and release electrical energy. Some of the highest energy experi- ments in the world use rooms full of charged capacitors to produce intense discharges.
As the charge on the capacitor increases, the electric field between the conductors in- creases, placing a growing stress on the insu- lator. At some critical point, the insulator breaks down and the capacitor ‘short circuits,’ releasing the stored electrical energy suddenly. Such breakdowns may destroy a solid insula- tor and with it, the capacitor. However, if the charging rate is slow and the insulator is air or liquid, the damage may ‘self-repair’ as fresh insulating material rushes in. If the current is strong or the insulator weak, current will pass
between the conducting plates, either steadily or in bursts. This is called a ‘leaky capacitor.’
Power transmission lines form large-scale capaci- tors with the air as insulator between the conducting wires. The geometry makes the electric field strong- est at the wire surface, which is where the air is like- liest to ‘break down’ and discharge. The hissing and crackling you hear when standing under a high- voltage power line is just this intermittent leakage.
Many natural systems form capacitors as well. For example, the Earth’s surface and its ionosphere are two conducting layers separated by air. The earth-ionosphere capacitor is of particular interest in the study of sprites. Small ‘leaks’ in the form of lightning can trigger much larger ‘leaks’ (sprites,
etc.) at high altitudes above them. In the electric uni- verse, this effect can be traced via auroral circuits, through the circuitry of the solar system, and far into interstellar space. From this viewpoint sprites and lightning are merely leakage currents trickling off the galactic power line. But clearly, the degree to which electric potential from the galaxy powers thunderstorms and affects climate on Earth has yet to be investigated.
Illustration of Earth’s role as a self-repairing, ‘leaky capacitor.’ The leakage of current across the insulating atmosphere appears as lightning, triggering further discharging above the clouds.
Credit: W. Thornhill
INFORMATION PANEL
language describing the formation of stars and galaxies includes many terms more familiar to electrical engineers than to astronomers or astrophysicists—Langmuir sheaths, z-pinches, glow discharges, arc discharges, plasmoids.
Having banished electric currents from space, astrophysicists are free to focus on the much simpler behavior of a magnetizable gas. The equations for the behavior of plasma are typically those used to describe flowing water and blowing wind, with a modification due to magnetic effects—the mathematics of classical mechanics. In fact, the assumptions are clear from the name chosen for the study of electrically inactive plasma: magnetohydrodynamics. And the new lexicon reflects the perspective, with words one might expect from a weatherman—winds and jets, bow shocks and shock waves, winds and rains of charged particles, wind socks, etc.
The difference between the two approaches is more than a simple choice of words. A plasma sheath is not the same thing as a bow shock. A bow shock will never reach a threshold of electrical imbalance and break down into the extraordinary configurations seen in the laboratory and deep space. And the implications reach far beyond our own planet. What NASA scientists call a “surprising rain of charged particles” in the vicinity of Jupiter’s moon Io is interesting and dangerous weather for spacecraft. But in an electric universe the phenomenon means much more than a cosmic weather report. It is a signature of electrical activity that could not fail to produce other surprises as well.